the Creative Commons Attribution 4.0 License.
the Creative Commons Attribution 4.0 License.
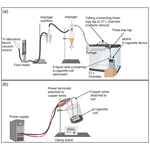
A rapid semi-quantitative screening method to assess chemicals present in heated e-liquids and e-cigarette aerosols
Natalie Anderson
Paul Pringle
Ryan Mead-Hunter
Benjamin Mullins
Alexander Larcombe
Sebastien Allard
Electronic cigarettes (e-cigarettes) lack regulatory status as therapeutic products in all jurisdictions worldwide. They are potentially unsafe consumer products, with significant evidence they pose a risk to human health. Therefore, developing rapid, economical test methods to assess the chemical composition of e-liquids in heated and unheated forms and the aerosols produced by e-cigarettes is crucial. Four different e-liquids were heated using two different methods: (1) “typical” vaping using an e-cigarette device, by cycling “on” for 3 s every minute for 2 h (e-liquid obtained from remainder in the tank and aerosol collected in an impinger), and (2) “accelerated” heating, using an e-cigarette coil submerged in e-liquid and heating in short 20 s bursts on then 20 s off for 2 min only (liquid traps aerosol produced). All e-liquids were then analysed to test for the presence and quantity of 13 chemicals by gas chromatography–mass spectrometry and compared to an unheated sample. E-liquids heated with the accelerated method showed a comparable trend to the typical heating method, i.e. increase or decrease in chemical compound quantity, for more than two-thirds of the detected compounds analysed over all e-liquids. Six chemicals were detected as aerosol from the impinger fluid with the typical heating method at negligible levels. We propose that this accelerated version of the typical vaping method could form the basis of a standardized screening tool to test heated e-liquids (and e-cigarette aerosols) for harmful or banned substances. This will ensure that only approved products reach the consumer and reduce potential e-cigarette harm.
- Article
(1677 KB) - Full-text XML
- BibTeX
- EndNote
Chemicals are present in the aerosol produced by electronic cigarettes (e-cigarette) when the e-liquid it contains is heated and aerosolized (Goniewicz et al., 2014; European Parliament, 2014). The e-cigarette refers to the aerosol-generating device, which uses the “e-liquid” to create aerosol by an evaporation–condensation method. If in sufficient quantities, and dependent on the hazard type, chemicals present in an e-cigarette-produced aerosol have the potential to negatively impact health when inhaled (European Association for the Co-ordination of Consumer Representation in Standardisation, 2021). For example, group 1 carcinogens acetaldehyde and formaldehyde have been found in e-cigarette aerosols and are degradation products of the e-liquid base components, propylene glycol and glycerol (Goniewicz et al., 2014). Other chemical ingredients found in unheated e-liquids that pose a risk to human health (e.g. respiratory irritants, sensitizers) may include flavours or solvents added to the e-liquids. These chemicals can be present in unheated e-liquid, heated e-liquid, aerosolized e-liquid, or any combination of the three forms. Yet, as recently as 2015, no country in the world regulated e-liquid ingredients beyond nicotine levels.
As more evidence emerges suggesting that e-cigarette aerosols negatively impact health, regulation is rapidly evolving in this area (European Parliament, 2014; Budzyńska et a., 2020; Therapeutic Goods Administration, 2021). For example, the European Union (EU) Tobacco Product Directive (TPD) states that only ingredients in nicotine-containing e-liquids that do not pose a risk to human health in heated or unheated forms can be used (European Parliament, 2014), and many countries (including United Kingdom, Germany, and France) banned some ingredients as a result (European Parliament, 2014; European Association for the Co-ordination of Consumer Representation in Standardisation, 2021; Budzyńska et al., 2020). A modest ingredient ban for nicotine-containing e-liquids came into effect in October 2021 in Australia, prior to a complete ban on non-prescription e-cigarette (and e-liquid) importation and sale in Australia being announced in May 2023 (Nogrady, 2023; Therapeutic Goods Administration, 2021). Known health effects of banned ingredients include e-cigarette- or vaping-associated pulmonary (or lung) injury (a.k.a VAPI or VALI/EVALI). There is emerging evidence of self-reported lung conditions associated with e-cigarette use (Winnall et al., 2023; Osei et al., 2020; Bircan et al., 2021). Health concerns are amplified by a multibillion-dollar market driving product sales and increasing use in young people. Consequently, pre-market approvals (including unheated ingredient listing), which allow independent determination of product safety (Australian Government, 2023; United States Food and Drug Administration, 2023), are preferred by regulators.
Whilst chemical testing of unheated e-liquids is relatively common, the safety of e-liquids remains largely unassessed due to the sheer scale of the market. Additionally, except for the EU TPD guidance (to the best of our knowledge), testing heated e-liquids for inclusion in pre-market product approval is not required in Australia or elsewhere (European Parliament, 2014; Winnall et al., 2023; Scientific Committee on Health Environmental and Emerging Risks, 2021; Larcombe et al., 2022). Methods to assess chemical content generally involve testing of unheated e-liquids or the e-cigarette aerosol generated, but not the heated e-liquid (Scientific Committee on Health Environmental and Emerging Risks, 2021). However, the heated e-liquid is more representative of what the user inhales compared to the unheated e-liquid and easier to assess when compared to e-cigarette aerosol (Larcombe et al., 2022; Erythropel et al., 2019). Importantly, chemicals present in e-liquids are known to degrade due to heating, by either boiling or evaporative convection (depending on wetted-wick temperature), and this can be exacerbated by the presence of catalytic surfaces such as Kanthal. The secondary products formed, at high (> 200 ∘C) or low temperatures (< 200 ∘C), may have increased or decreased toxicity compared to the parent compound (Erythropel et al., 2019; Floyd et al., 2019; Goniewicz et al., 2014; Jaegers et al., 2021; Li et al., 2021; Zhao et al., 2016; Saliba et al., 2018).
Many approaches have been trialled to address the difficulties in directly assessing the chemical composition of e-cigarette-produced aerosol. Such challenges include collecting enough aerosol to perform an assay (and therefore detect potentially toxic compounds) and overload of the main excipients (propylene glycol and vegetable glycerin). These difficulties induce increasingly complicated test methods, yet current methods have been shown to be outdated or limited (Floyd et al., 2019; Herrington and Myers, 2015; Scientific Committee on Health Environmental and Emerging Risks, 2021). Despite the expansive range of tests, a simple test capable of assessing heated and unheated e-liquids, and the aerosol produced all at once, is yet to be established, but vital.
In this study we aimed to (1) establish and validate a simple “accelerated”, method of heating and aerosolizing e-liquids (i.e. “vaping”) that would be comparable to, but quicker than, the “typical” method of heating and aerosolizing e-liquid and (2) assess the chemical composition of the heated e-liquid and the aerosol produced all at once. We hypothesized that the accelerated method and the commonly used typical vaping method would result in similar heating-induced chemical changes in the e-liquids. The two heating methods were compared by measuring the presence and concentration of a range of chemicals. We hope that this accelerated test methodology can form the critical first step in establishing a rapid test for screening of e-liquids for banned substances.
2.1 Typical vaping process
A set-up was designed to replicate the heating–cooling process an e-liquid would undergo when an e-cigarette is used in a typical way (Fig. 1a) (Etter and Bullen, 2014; St Helen et al., 2016; CORESTA, 2015). The method allowed sample collection at two points for analysis of heated e-liquid and aerosol, respectively: (1) from the remainder in the e-liquid tank (atomizer) and (2) from the impinger (Fig. 1a). To begin, the e-cigarette (MVP4, Innokin, Shenzhen, China, operating wattage range of 6–100 W, temperature range of 150–315 ∘C, maximum current of 35.5 A) atomizer (the e-liquid tank, containing e-cigarette coil) was filled with ∼ 3.5 mL of e-liquid, and the impinger was filled with 5 mL of e-liquid excipient (50 : 50 glycerin–propylene glycol (), Sigma Aldrich, Milwaukee WI, USA). A flow of ∼ 3 L min−1 ambient filtered air was drawn through the system via a laboratory bench vacuum and kept stable through monitoring with a flow meter (max 5 L min−1, TSI, 800669, Shoreview, MN). New coils (Kanthal BVC, 100–200 W, 0.28 Ω, Innokin Scion) were used each time to avoid cross-contamination of chemical species and to control for coil ageing effects. The e-cigarette device was set to 80 W (reading 0.28–0.35 Ω) each time the device was connected to the atomizer. To vape the device in line with recommendations by the Cooperation Centre for Scientific Research Relative to Tobacco (CORESTA), the ignition button was held for ∼ 3 s, and the aerosol was drawn from the device into a 60 mL syringe and then expelled through two ∼ 4 mm ID, 15 cm tubing lengths into the custom-made (27 L, 30 × 30 × 30 cm) chamber using a three-way tap (Dispoflex™, Disposafe Health and Lifecare Ltd, Haryana, India) (CORESTA, 2015). This process was repeated every minute for 2 h (with the atomizer tank refilled after ∼ 60 min), for 120 puffs total. While we acknowledge that vaping topography is extremely variable, 120 puffs over a 2 h period (120 puffs × 60 mL, therefore 7.2 L of inhaled aerosol-containing air) was chosen to be representative of what a typical vaper might use (Etter and Bullen, 2014). After heating, the liquid was transferred to glass sample vials and kept at 4 ∘C to minimize the loss of volatiles.
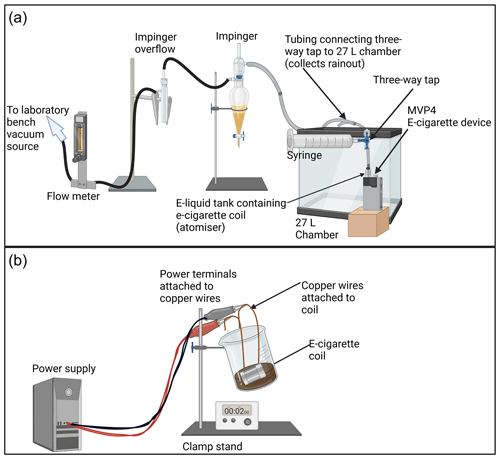
Figure 1Vaping set-ups. (a) Typical vaping set-up. A vacuum drew air through the system at ∼ 3 L min−1. The aerosol was drawn into a 60 mL syringe, and a three-way tap was turned to allow the syringe to push the aerosol through two ∼ 4 mm i.d. and ∼ 15 cm tubing lengths and into the 27 L chamber for mixing. Air containing aerosol was drawn first into an impinger with 50 : 50 propylene glycol–glycerin base liquid. (b) Accelerated vaping set-up. The power supply was attached to copper wires, which were attached to an e-cigarette coil (Kanthal BVC), which was always submerged in e-liquid within a 100 mL beaker. Key differences between methods: (1) aerosol is allowed to mix in air before capture rather than impinged immediately in liquid, and (2) volume of liquid is different; 3.5 mL is present in the atomizer with the typical method compared to ∼ 30 mL in the beaker with the accelerated method. Key similarities between methods: both methods apply the same amperage to the coil (and therefore heat coil to the same temperature). Created with https://www.biorender.com/ (last access: 21 October 2023).
2.2 Accelerated vaping process
An accelerated vaping process was developed, based on standard tests for oxidation of oils (Fig. 1b) (American Society for Testing Materials, 2022). Our premise for collection of e-cigarette aerosols in the liquid was as follows:
-
An e-cigarette is an evaporation–condensation aerosol generator, intended to modify the e-liquid as little as possible during aerosolization; however, it does thermo-oxidize, hence the need for this research.
-
Our accelerated method of heating the e-liquid via a submerged coil creates a “bubbling aerosol generator” (Vidmantas et al., 1997). Like an evaporation aerosol condensation generator, a bubbling generator will modify the e-liquid minimally; however, it may allow more volatile compounds to preferentially aerosolize.
-
The creation of an aerosol via bubbling can allow aerosol capture whilst bubbling either through the bulk liquid (when cooling) or at the gas–liquid surface (Ghiaassiaan and Yao, 1997; Koch and Weber, 2012).
-
Surface bubbles can generate aerosol by either jet or film droplets when they burst, and based on combinations of surface tension and bubble size, aerosol will recombine with the liquid the bubble arises from when it bursts (Koch and Weber, 2012; Mead-Hunter et al., 2018).
-
Thereby, through a combination of these processes it is reasonable to assume we retain a representative sample of the same material that is aerosolized, as well as possibly more of the thermo-oxidized (aged) material we are interested in.
To create the bubbler, an identical Kanthal BVC coil, as used in the typical vaping process, was connected to a power supply (MP3090, PowerTech, China) by means of solid coper wires connected to each end of the coil (end cap removed). The power supply was set at 7.4 V and 27 A (0.274 Ω) to stay within the maximum power (200 W) of the coil used for the typical process and to ensure that the resistance matched that of the typical vaping process. The coil was placed in a 100 mL beaker, which was open to air and held at a 45∘ angle with a clamp stand, and ∼ 30 mL of e-liquid was poured into the beaker, enough to completely submerge the coil and ensure the full volume of liquid would not heat to boiling temperature within the 1 min total heating period (20 s on 20 s off × 3), and limited planar surface evaporation would occur (Fig. 1b). The 45∘ angle was used both to minimize the liquid volume needed to immerse the coil and to ensure any aerosol (or vapour) produced would recondense on the wall of the beaker, allowing it to be collected for sampling. The power supply was then turned on to operate the coil for 5 × 20 s “burst” intervals, with 20 s pauses interspersed for a total “on” time of 1 min, mimicking a “short cluster” vaping pattern for a user (St Helen et al., 2016). After heating, the liquid was transferred to glass sample vials and kept at 4 ∘C to minimize the loss of volatiles.
2.3 Sample and chemical selection
Four flavoured e-liquids, labelled “nicotine-free”, were assessed – “butterscotch tobacco”, “menthol”, “choc caramel”, and “tiramisu” – which were purchased from online suppliers and analysed as 50 : 50 propylene glycol–glycerin () ratios. The propylene glycol–glycerol mixture was selected as 50 : 50 since it is a commonly sold ratio. Each e-liquid chemical composition was assessed using both methods to quantify 13 chemicals – 4-(4-methoxyphenyl)-2-butanone, ethyl vanillin, eugenol, nicotyrine, nicotine, menthol, thymol, ethyl maltol, trans-cinnamaldehyde, 2-chlorophenol, benzyl alcohol, benzaldehyde, and furfural – with a molecular weight range from 178.23 to 96.09 g mol−1. The 13 chemicals were chosen based on (i) being previously identified, known ingredients in e-liquids and (ii) the availability of a standard for the chemical (Larcombe et al., 2022).
2.4 Chemical analysis method of accelerated and typical vaping process
Thirteen chemicals were tested for, in four different e-liquids, using gas chromatography–mass spectrometry. For each of the four e-liquids, we tested for chemicals in three forms – (i) “unheated” e-liquid (i.e. straight out of the bottle), (ii) remainder of e-liquid in the atomizer and collected from the impinger after typical vaping, and (iii) e-liquid remaining in the beaker after accelerated vaping. The latter sample (iii) was taken in order to detect aerosols, assuming that aerosols (not vapour) would be captured in the e-liquid during the accelerated method. The aerosol generated from the typical method was captured in an impinger containing 50 : 50 () glycerin–propylene glycol. Our intention was for this collected aerosol in the impinger to be added to the atomizer tank sample for equivalent comparison to the accelerated sample; however negligible values for the impinger result meant that they were excluded from the final analysis.
Samples obtained from both methods used to heat e-liquids were compared to unheated e-liquids, both within e-liquid type and within chemical compound, with the purpose of the comparison being to identify trends of increase or decrease from unheated e-liquid.
2.5 Chemical detection and analysis
Chemical analysis of accelerated and typical vaping process e-liquids, including sample and chemical detection, has been previously described in detail elsewhere (Larcombe et al., 2022). The samples (0.25 g) were accurately weighed and placed into amber vials with 4.75 mL ultrapure water. Thereafter, 10 µL of a 1 g L−1 4-bromophenol-d4 stock solution was added as an internal standard. Prior to the analysis, 1.6 g of analytical-grade sodium chloride was added to increase volatilization and the vials tightly capped. To facilitate adsorption, the samples were incubated at 90 ∘C for 15 min prior to solid-phase micro-extraction using a divinylbenzene/carboxen/polydimethylsiloxane fibre from Supelco®, allowing for 13.6 min adsorption of the analytes on the fibre. The fibre was then desorbed at 250 ∘C in the injector in spitless mode for 5 min followed by 15 min in split mode. A Gerstel MPS2 multifunction autosampler was used to perform automated solid-phase micro-extraction injections. Analysis was carried out with an Agilent 6890N gas chromatograph interfaced with an Agilent 5973 network mass selective detector, fitted with a HP-INNOWax polyethylene glycol stationary-phase capillary column (30 m, 0.25 mm, 0.25 µm, Agilent J&W, Australia), to separate polar compounds. A constant flow (1.2 mL min−1) of helium (99.999 % pure, BGC, Australia) was used as a carrier gas. Optimal gas chromatography–mass spectrometry conditions were determined, as measured by maximum sensitivity, baseline separation of analytes, and Gaussian peak shapes. In order to ensure a good separation of the different analytes, the oven was held isothermal at 37 ∘C (2 min), then heated to 260 ∘C at 5 ∘C min−1 and held at the final temperature for 10 min. Detection of analytes was carried out using a mass spectrometer in electron impact ionization mode at 70 eV. The mass spectrometer quadrupole temperature was set at 150 ∘C and the mass spectrometer source at 230 ∘C. The compounds were identified using a combination of their retention times and comparison of the mass spectra data of pure compounds and the specific diagnostic ion fragments of each component, with the National Institute of Standards and Technology Mass Spectral search programme from the NIST (National Institute of Standards and Technology)/EPA (Environmental Protection Agency)/NIH EI (National Institutes of Health Electron Ionization Library) and NIST Tandem Spectral Library, which came integrated with the analysis software.
Over all e-liquids, 3 of the 13 compounds tested for were not detected in any e-liquid form (4-(4-methoxyphenyl)-2-butanone, thymol, 2-chlorophenol) (Table 1).
Table 1Assessment of 13 different chemical compounds from four e-liquids in both unheated and heated samples (accelerated and typical vaping methods). The unheated sample is comprised of only e-liquid in “fresh” or un-vaped form. The heated sample for the typical method assessed the leftover from the atomizer tank, and the accelerated method assessed heated e-liquid produced from a coil submerged in e-liquid. Chemicals are listed in alphabetical order. Bold font: increase from unheated sample. Acc: accelerated or aged. U: undetected. Italicized results indicate that the compound was not present in one of the heating method samples, and therefore fold change analysis was not possible for that chemical in that e-liquid flavour. Numbers are accurate to three significant figures (digits), and values less than zero are displayed with logarithmic scale for ease of reading.
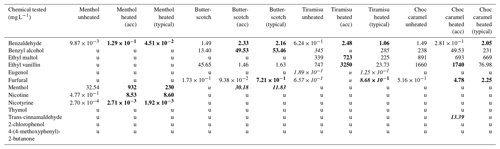
3.1 Inclusion and exclusion criteria for analysis
There were 16 instances where a chemical was detected in unheated and both heated forms (Table 1), and all were included in analysis. Analysis involved (1) simple comparison in table format of the heated (two methods) and unheated form of an e-liquid sample and (2) comparison via fold change compared to unheated (, where X is the unheated sample (mg L−1 concentration), and Y is the heated sample (mg L−1 concentration)) for both typical and accelerated heating methods.
Fold-change analyses were not possible on the following: one chemical was undetected in unheated form but detected in both heated forms (menthol in butterscotch tobacco); one chemical was undetected in unheated form and detected in only one heated form (trans-cinnamaldehyde in choc caramel). A further three chemicals were detected in unheated form and only one heated form (benzyl alcohol (tiramisu), eugenol (tiramisu), and furfural (tiramisu)) (Table 1, represented by italicized values).
3.2 Behaviour of the different chemicals detected in e-liquids
To compare the effect of heating, results are displayed as fold change compared to unheated (, where X is the unheated sample (mg L−1 concentration), and Y is the heated sample (mg L−1 concentration)) for both typical and accelerated heating methods (Fig. 2). Specific chemicals (benzaldehyde, benzyl alcohol, ethyl vanillin, ethyl maltol, furfural, menthol, nicotine, and nicotyrine) were present in unheated form and both heated forms in 16 instances.
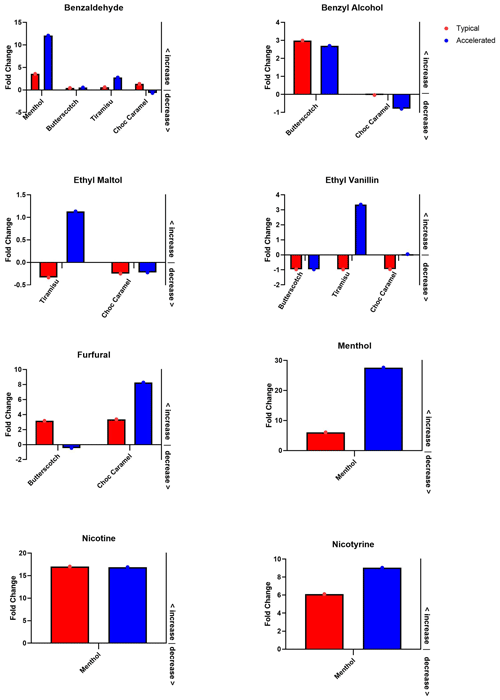
Figure 2Fold change comparison between heating methods. E-liquid flavours are described on the x axis. Accelerated and typical methods are indicated by the blue and red, respectively. The y axis indicates the fold change compared to unheated, i.e. fold change = , where X is the unheated sample (mg L−1 concentration), and Y is the heated sample (mg L−1 concentration). Note different scales. Values that fall below the horizontal line at zero indicate a decrease in concentration from the unheated sample; values above zero are an increase from unheated. If any chemical was not detected in a particular flavour then those flavours are not shown (e.g. there was no ethyl maltol detected in the menthol or butterscotch flavour). Graph created with GraphPad Prism 8.
Over all e-liquids, in these 16 instances, 70 % () demonstrated a consistent trend within chemical type; i.e. both methods of heating either increased or decreased in concentration compared to the unheated sample. Ethyl vanillin (choc caramel and tiramisu), furfural (butterscotch tobacco), ethyl maltol (tiramisu), and benzaldehyde (choc caramel) are the five exceptions.
3.3 Chemical characterization by e-liquid type
In the menthol e-liquid, of the 13 chemicals tested, 9 were not detected in the heated or unheated sample (Table 1). Of the four that were detected (nicotine, nicotyrine, menthol, and benzaldehyde), all (, 100 %) exhibited the same trend (increasing concentration) after heating when compared to the unheated sample for both typical and accelerated heating methods (Table 1, Fig. 2).
In the butterscotch tobacco e-liquid, of 13 chemicals tested, 8 were not detected in heated or unheated form, and an additional 1 (menthol) was undetected in unheated form (Table 1). Of the four chemicals detected in each sample, three (, 75 %) (ethyl vanillin, benzyl alcohol, and benzaldehyde) exhibited the same trend for both typical and accelerated heating methods when compared with the unheated e-liquid (Table 1, Fig. 2). However, furfural increased after typical heating but decreased with the accelerated method when compared to the unheated sample.
In the tiramisu e-liquid, of 13 chemicals tested, 7 were not detected in heated or unheated form, and an additional 3 were undetected in 1 form of heating (Table 1). Of the three detected in each sample, one (, 33 %) (benzaldehyde) exhibited the same trend after both typical and accelerated heating (Table 1, Fig. 2). The remaining two chemicals detected (ethyl vanillin and ethyl maltol) both decreased after typical heating but increased after accelerated heating when compared to the unheated sample.
In the choc caramel e-liquid, of 13 chemicals tested, 7 were not detected in heated or unheated form, and an additional 1 (trans-cinnamaldehyde) was undetected in both unheated and heated form (Table 1). Of the five chemicals detected in each sample, three (, 60 %) (benzyl alcohol, ethyl maltol, and furfural) exhibited the same trend after both typical and accelerated heating methods (Table 1, Fig. 2). Benzaldehyde increased after typical heating but decreased after accelerated heating compared to the unheated sample. Conversely, ethyl vanillin increased after accelerated heating but decreased after typical heating when compared to the unheated sample.
3.4 Impinger results from typical vaping heating method
Only 6 of the 13 chemicals tested were detected at negligible quantities in the impinger fluid – furfural, benzaldehyde, menthol, benzyl alcohol, ethyl maltol, and ethyl vanillin – and as such they were unable to be included in final analysis.
The accelerated method used here is simple and cost-effective and has the potential to produce heated e-liquid and aerosol in a similar manner to an e-cigarette but on an accelerated time frame, allowing chemical assessment in a single experiment. Due to the prohibitive costs of commercially available vaping machines, many “in-house” simplified methods and set-ups (e.g. e-cigarette puffing machines) have been developed. However, to the best of our knowledge, they all focus on generation of e-cigarette aerosol and not on assessment of the heated e-liquid (Palazzolo et al., 2021).
Comparison of the accelerated and typical heated samples with their unheated counterpart showed that in ∼ 70 % of the e-liquids tested the heating methods demonstrated a similar trend, i.e. increase or decrease in chemical concentration. The four chemicals implicated in the five differing comparisons were mostly aldehydes (ethyl vanillin (), furfural (), and benzaldehyde ()) except for ethyl maltol (), being an alcohol. In two chemicals – ethyl vanillin () and ethyl maltol () – an increase in chemical concentration with the accelerated heating method compared to the typical method was detected. The observed “increase” with the accelerated method can be attributed to a loss of aerosol with the typical vaping experimental method. Rainout (recondensation of the aerosol as it cools) of the liquid aerosol was observed in the three-way tap system and the very thin tubing (ID ∼ 4 mm) connecting the tap system to the 27 L chamber (Fig. 1a). A modification of the design (e.g. larger tubing) would reduce the rainout losses, thus increasing the yield from the impinger with the typical method and improving reproducibility between methods. It is also possible that the flavour aldehydes were present in their propylene glycol acetal form instead of their aldehyde form, as aldehydes are known to form acetals readily (Erythropel et al., 2019). The inability to fully capture the aerosol from the typical heating method due to rainout meant we are unable to confirm the suitability of our accelerated heating method as an impinger for aerosol, but only its validity to compare heating methods.
The remaining two discrepancies involved furfural () and benzaldehyde (), and these compounds were found in increased quantities with the typical method compared to the accelerated method. Because these two discrepancies contain low-molecular-weight and low-boiling-point products we suspect they may have evaporated more readily (compared to the typical method) and that our accelerated method was simply unable to capture compounds with low boiling point that volatilize easily (Erythropel et al., 2019). For example, furfural has the lowest boiling point and molecular weight of all chemicals detected (162 ∘C, 96.09 g mol−1) and was detected to have decreased from the unheated sample in both butterscotch and tiramisu flavours. While our study design angled the beaker at 45∘ to allow re-condensation of any vapour on the beaker wall, the experiment was carried out in a ventilated fume hood, which may have increased the loss of highly volatile compounds. In future studies, the addition of a lid on the angled beaker as well as monitoring the liquid temperature on the wick (or surrounding the wick) may help to reduce discrepancies to allow full validation of the method for detection of aerosols (Li et al., 2021; Palazzolo et al., 2021; Bitzer et al., 2018). Further studies should also be designed to consider the solubility of the flavouring compound in the base excipients (i.e. propylene glycol or glycerol) and not the boiling point, as this is suggested to be a major indicator of whether a compound will be detected in and therefore carried over into an aerosol (Erythropel et al., 2019).
Undetected chemicals included 4-(4-methoxyphenyl)-2-butanone, thymol, and 2-chlorophenol. Considering that no “fruity” flavours were assessed, it is less surprising that 4-(4-methoxyphenyl)-2-butanone was undetected, as it is a raspberry ketone methyl ether – a common flavouring in “berry”-flavoured e-liquids. Although we were looking for thymol because of its use as a precursor for racemic menthol (produced from m-cresol), its absence may be explained because pulegone and other terpenoids are also used as the precursor (Dylong et al., 2022). Additionally, menthol was not detected in either tiramisu or choc caramel flavours, so perhaps synthetic analogues were present (where menthol was not detected) such as N-ethyl 2-isopropyl-5-methylcyclohexanecarboxamide (trade name WS-3). As the demand for menthol increases, alternative methods to produce L-menthol are on the rise, such as from citronellal (Dylong et al., 2022).
Chlorophenols like 2-chlorophenol have previously been detected in e-liquids probably because they are notorious environmental contaminants. Particularly, 2-chlorophenol is a priority contaminant in both the US and EU and has previously been found in e-liquids (Larcombe et al., 2022; Chivers et al., 2019; Igbinosa et al., 2013); 2-chlorophenol is used for many applications, predominantly as a detergent but also as an intermediate in the manufacturing of agricultural chemicals, pharmaceuticals, biocides, and dyes. Therefore, it is commonly detected in environmental water samples after being discharged from industrial effluents (Igbinosa et al., 2013; Yahaya et al., 2019). It has previously been suggested that 2-chlorophenol may be a contaminant from the glycerin excipient for two reasons: (1) vegetable glycerin is made from plant crops such as canola, and 2-chlorophenol has been found in canola as a pesticide residue, and (2) glycerin (not from plants) is a by-product of biodiesel production, and biodiesel can be made with canola (Abdel-Gawad and Hegazi, 2010; Yahaya et al., 2019). It is possible that derivatives of 2-chlorophenol or other phenolic derivatives known to be priority contaminants were present. However, it was not within the scope of the study to assess these compounds as they are not commonly reported to be found and/or tested for in e-liquids. Eugenol and trans-cinnamaldehyde were the least detected compounds; they were found in only one flavour e-liquid. Cinnamaldehyde might not be detected because it is known to form propylene glycol acetals (αβ, unsaturated aldehyde), and this form is known to be relatively reactive (Erythropel et al., 2019).
Limitations and future directions
A limitation of this study is that we tested a pre-determined list of chemicals, based on our knowledge of known e-liquid ingredients, available standards, and available analytical methods, rather than looking for a complete chemical characterization. This approach allowed us to test a larger range of e-liquids and demonstrate the utility of the accelerated ageing technique, as per the overarching goal of the study. However, an “open-ended” approach may be useful for future studies if this method is to become standardized. An open-ended approach would allow a more complete comprehension of the ageing process and oxidation reactions occurring but would require a broader range of analytical techniques than demonstrated here. Furthermore, whilst we assessed for some ingredients which are now banned (i.e. benzaldehyde and cinnamaldehyde), this study was designed and conducted prior to the enactment of banned ingredients by the Therapeutic Goods Administration in 2021 (Therapeutic Goods Administration, 2021), and expanding future analysis to include the full range of banned chemical products would be helpful. The same comment applies for chemicals banned in other jurisdictions.
The method we describe in this study has many advantages over current methods for testing e-liquids. It is a rapid and inexpensive set-up allowing assessment of the chemical composition of heated e-liquids and, with minor modifications, their resultant aerosols. It could be used with any available coil that can be modified and powered as described. Furthermore, the accelerated method is likely to capture aerosol generated from a heated e-liquid in a manner comparable to the “typical user” vaping method as described in CORESTA in terms of both type and quantity of chemicals produced. Our submerged, rapid heating–cooling method can economically sample heated liquid and aerosols (but not vapour) within a single sample in 2 min, which may have advantages over some other methods. This method is more representative of what the user inhales as it is testing a heated liquid during exposure to the coil (potentially) catalytic surface rather than only an unheated e-liquid.
In summary, the accelerated method described here is a suitable screening tool for rapid chemical assessment of heated e-liquids and their aerosols that mimics typical e-cigarette vaping on an accelerated time frame. The EU TPD recommendation is to assay heated e-liquids; however (to the best our knowledge) there has only been one previously published study on the effects of ageing and/or heating on e-liquids. We propose that this method (with our recommended improvements) can be used as a standardized screening tool for e-liquids and their aerosols to identify potentially harmful chemicals, such as those recently banned in Australia or previously banned in Europe and the United Kingdom. With minor modification, this test could be used prior to importation or sale to ensure that only tested products, containing approved ingredients, reach the consumer. In the absence of an approved therapeutic goods status for e-cigarettes, the type of high-throughput testing described here is necessary as a minimal precaution to assess and reduce the potential harms of a consumer product that is generally accepted in the public to be a less harmful alternative to smoking.
Data can be obtained upon request by contacting the author.
NA: data curation, formal analysis, investigation, methodology, project administration, visualization, writing (review and editing), writing (original draft). PP: data curation, formal analysis, investigation, methodology, validation, writing (review and editing). RMH: formal analysis, investigation, writing (review and editing). BM: conceptualization, methodology, resources, writing (review and editing), funding acquisition. AL: conceptualization, methodology, project administration, resources, supervision, visualization, writing (review and editing), funding acquisition. SA: data curation, formal analysis, investigation, methodology, resources, supervision, writing (review and editing), validation, visualization.
The contact author has declared that none of the authors has any competing interests.
Publisher’s note: Copernicus Publications remains neutral with regard to jurisdictional claims made in the text, published maps, institutional affiliations, or any other geographical representation in this paper. While Copernicus Publications makes every effort to include appropriate place names, the final responsibility lies with the authors.
This work was supported by funding from the Minderoo Foundation, Lung Foundation Australia, Cancer Council WA, and the Scottish Masonic Charitable Foundation.
This research has been supported by the Minderoo Foundation and the Scottish Masonic Charitable Foundation (grant no. CTR-JL-14357-1), the Lung Foundation Australia (grant no. CTR-JL-14356-1), and Cancer Council WA.
This paper was edited by Mar Viana and reviewed by Snezana Radivojev and one anonymous referee.
Abdel-Gawad, H. and Hegazi, B.: Fate of 14C-ethyl prothiofos insecticide in canola seeds and oils, J. Environ. Sci. Heal. B, 45, 116–122, https://doi.org/10.1080/03601230903471878, 2010.
American Society for Testing Materials: Standard test method for bench oxidation of engine oils by ROBO apparatus D7528-09, American Society for Testing Materials, https://doi.org/10.1520/D7528-22, 2022.
Australian Government, Department of Health and Aged Care, Therapeutic Goods Administration: Proposed reforms to the regulation of nicotine vaping products, https://consultations.tga.gov.au/medicines-regulation-division/proposed-reforms-to-the-regulation-of-nicotine-vap/, last access: 21 July 2023.
Bircan, E., Bezirhan, U., Porter, A., Fagan, P., and Orloff, M. S.: Electronic cigarette use and its association with asthma, chronic obstructive pulmonary disease (COPD) and asthma- COPD overlap syndrome among never cigarette smokers, Tob. Induc. Dis., 19, 1–9, https://doi.org/10.18332/tid/132833, 2021.
Bitzer, Z. T., Goel, R., Reilly, S. M., Foulds, J., Muscat, J., Elias, R. J., and Richie Jr., J. P.: Effects of Solvent and Temperature on Free Radical Formation in Electronic Cigarette Aerosols, Chem. Res. Toxicol., 31, 4–12, https://doi.org/10.1021/acs.chemrestox.7b00116, 2018.
Budzyńska, E., Sielemann, S., Puton, J., and Surminski, A. L. R. M.: Analysis of e-liquids for electronic cigarettes using GC-IMS/MS with headspace sampling, Talanta, 209, 120594, https://doi.org/10.1016/j.talanta.2019.120594, 2020.
Chivers, E., Janka, M., Franklin, P., Mullins, B., and Larcombe, A.: Nicotine and other potentially harmful compounds in “nicotine-free” e-cigarette liquids in Australia, Med. J. Australia, 210, 127–128, https://doi.org/10.5694/mja2.12059, 2019.
Cooperation Centre for Scientific Research Relative to Tobacco (CORESTA): CORESTA recommended method No. 81. Routine Analytical Machine for E-Cigarette Aerosol Generation and Collection – Definitions and Standard Conditions, ISA 7210:2013, https://www.coresta.org/sites/default/files/technical_documents/main/CRM_81.pdf (last access: 21 October 2023), 2015.
Dylong, D., Hausoul, P. J. C., Palkovits, R., and Eisenacher, M.: Synthesis of (-)-menthol: Industrial synthesis routes and recent development, Flavour Frag. J., 37, 195–209, https://doi.org/10.1002/ffj.3699, 2022.
Erythropel, H. C., Jabba, S. V., DeWinter, T. M., Mendizabal, M., Anastas, P. T., Jordt, S. E., and Zimmerman, J. B.: Formation of flavorant-propylene Glycol Adducts With Novel Toxicological Properties in Chemically Unstable E-Cigarette Liquids, Nicotine Tob. Res., 21, 1248–1258, https://doi.org/10.1093/ntr/nty192, 2019.
Etter, J.-F. and Bullen, C.: A longitudinal study of electronic cigarette users, Addict. Behav., 39, 491–494, 2014.
European Association for the Co-ordination of Consumer Representation in Standardisation (ANEC): Position Paper: e-cigarettes and e-liquids – limits for chemicals – basis for discussion, European Association for the Co-ordination of Consumer Representation in Standardisation (ANEC), https://www.anec.eu/images/Publications/position-papers/Chemicals/ANEC-PT-2019-CEG-005.pdf (last access: 25 October 2023), 2021.
European Parliament: Directive 2014/40/EU of the European Parliament and of the Council of 3 April 2014 on the approximation of the laws, regulations and administrative provisions of the member states concerning the manufacture, presentation and sale of tobacco and related products and repealing Directive 2001/37/EC, Off. J. Eur. Union, 57, L127/121–L127/138, 2014.
Floyd, E. L., Queimado, L., Wang, J., Regens, J. L., and Johnson, D. L.: Electronic cigarette power affects count concentration and particle size distribution of vaping aerosol, PLOS ONE, 13, e0210147, https://doi.org/10.1371/journal.pone.0210147, 2019.
Ghiaasiaan, S. M. and Yao, G. F.: A Theoretical Model for Deposition of Aerosols in Rising Spherical Bubbles due to Diffusion, Convection, and Inertia, Aerosol Sci. Tech., 26, 141–153, https://doi.org/10.1080/02786829708965420, 1997.
Goniewicz, M. L., Knysak, J., Gawron, M., Kosmider, L., Sobczak, A., Kurek, J., Prokopoqicz, A., Jablonska-Czapla, M., Rosik-Dulewska, C., Havel, C., Jacob III, P., and Benowitz, N.: Levels of selected carcinogens and toxicants in vapour from electronic cigarettes, Tob. Control, 23, 133–139, https://doi.org/10.1136/tobaccocontrol-2012-050859, 2014.
Herrington, J. S. and Myers, C.: Electronic cigarette solutions and resultant aerosol profiles, J. Chromatogr. A, 1418, 192–199, https://doi.org/10.1016/j.chroma.2015.09.034, 2015.
Igbinosa, E. O., Odjadjare, E. E., Chigor, V. N., Igbinosa, I. H., Emoghene, A. O., Ekhaise, F. O., Igiehon, N. O., and Idemudia, O. G.: Toxicological profile of chlorophenols and their derivatives in the environment: the public health perspective, Sci. World J., 2013, 460215–460215, https://doi.org/10.1155/2013/460215, 2013.
Jaegers, N. R., Hu, W., Weber, T. J., and Hu, J. Z.: Low-temperature (< 200 ∘C) degradation of electronic nicotine delivery system liquids generates toxic aldehydes, Sci. Rep.-UK, 11, 7800, https://doi.org/10.1038/s41598-021-87044-x, 2021.
Koch, D. and Weber, A. P.: Separation of gas-borne nanoparticles in bubble columns, J. Aerosol Sci., 53, 61–75, https://doi.org/10.1016/j.jaerosci.2012.05.012, 2012.
Larcombe, A., Allard, S., Pringle, P., Mead-Hunter, R., Anderson, N., and Mullins, B.: Chemical analysis of fresh and aged Australian e-cigarette liquids, Med. J. Aust., 216, 27–32, https://doi.org/10.5694/mja2.51280, 2022.
Li, Y., Burns, A. E., Tran, L. N., Abellar, K. A., Poindexter, M., Li, X., Madl, A. K., Pinkerton, K. E., and Nguyen, T. B.: Impact of e-Liquid Composition, Coil Temperature, and Puff Topography on the Aerosol Chemistry of Electronic Cigarettes, Chem. Res. Toxicol., 34, 1640–1654, https://doi.org/10.1021/acs.chemrestox.1c00070, 2021.
Mead-Hunter, R., Gumulya, M. M., King, A. J. C., and Mullins, B. J.: Ejection of Droplets from a Bursting Bubble on a Free Liquid Surface-A Dimensionless Criterion for “Jet” Droplets, Langmuir, 34, 6307–6313, https://doi.org/10.1021/acs.langmuir.8b00664, 2018.
Nogrady, B.: Australia bans all vapes except on prescription to stem use in children, BMJ Open, 381, 1014, https://doi.org/10.1136/bmj.p1014, 2023.
Osei, A. D., Mirbolouk, M., Orimoloye, O. A., Dzaye, O., Uddin, S. M. I., Benjamin, E. J., Hall, M. E., DeFilippis, A. P., Bhatnagar, A., Biswal S. S., and Blaha, M. J.: Association Between E-Cigarette Use and Chronic Obstructive Pulmonary Disease by Smoking Status: Behavioral Risk Factor Surveillance System 2016 and 2017, Am. J. Prev. Med., 58, 336–342, https://doi.org/10.1016/j.amepre.2019.10.014, 2020.
Palazzolo, D. L., Caudill, J., Baron, J., and Cooper, K.: Fabrication and Validation of an Economical, Programmable, Dual-Channel, Electronic Cigarette Aerosol Generator, Int. J. Env. Res. Pub. He., 18, 13190, https://doi.org/10.3390/ijerph182413190, 2021.
Saliba, N. A., El Hellani, A., Honein, E., Salman, R., Talih, S., Zeaiter, J., and Shihadeh, A.: Surface Chemistry of Electronic Cigarette Electrical Heating Coils: Effects of Metal Type on Propylene Glycol Thermal Decomposition, J. Anal. Appl. Pyrol., 134, 520–525, https://doi.org/10.1016/j.jaap.2018.07.019, 2018.
Scientific Committee on Health Environmental and Emerging Risks (SCHEER): Scientific Opinion on electronic cigarettes, Scientific Committee on Health Environmental and Emerging Risks (SCHEER), https://health.ec.europa.eu/system/files/2022-08/scheer_o_017.pdf (last access: 21 October 2023), 2021.
St Helen, G., Ross, K. C., Dempsey, D. A., Havel, C. M., Jacob, P., and Benowitz, N. L.: Nicotine Delivery and Vaping Behavior During ad Libitum E-cigarette Access, Tob. Regul. Sci., 2, 363–376, https://doi.org/10.18001/TRS.2.4.8, 2016.
Therapeutic Goods Administration: Therapeutic Goods Standard for Nicotine Vaping Products TGO 110 Order, Therapeutic Goods Administration, https://www.legislation.gov.au/Details/F2021L00595 (last access: 21 October 2023), 2021.
United States Food and Drug Administration: Premarket Tobacco Product Applications, https://www.fda.gov/tobacco-products/market-and-distribute-tobacco-product/premarket-tobacco-product-applications, last access: 21 July 2023.
Vidmantas, U., Willeke, K., Grinshpun, S. A., Donnelly, J., Lin, X., and Mainelis, G.: Aerosolization of Particles from a Bubbling Liquid: Characteristics and Generator Development, Aerosol Sci. Tech., 26, 175–190, https://doi.org/10.1080/02786829708965423, 1997.
Winnall, W. R., Greenhalgh, E. M., Bayly, M., and Scollo, M. M.: 18.5 Chemicals in e-liquids and e-cigarette aerosols, in: Tobacco in Australia: Facts and issues, edited by: Greenhalgh E. M., Scollo, M. M., and Winstanley, M. H., Cancer Council Victoria, Melbourne, https://www.tobaccoinaustralia.org.au/chapter-18-e-cigarettes/18-5-chemicals-in-e-liquids-and-e-cigarette-aerosols (last access: 21 October 2023), 2023.
Yahaya, A., Okoh, O. O., Agunbiade, F. O., and Okoh, A. I.: Occurrence of phenolic derivatives in Buffalo River of Eastern Cape South Africa: Exposure risk evaluation, Ecotox. Environ. Safe., 171, 887–893, https://doi.org/10.1016/j.ecoenv.2019.01.037, 2019.
Zhao, T., Shu, S., Guo, Q., and Zhu, Y.: Effects of design parameters and puff topography on heating coil temperature and mainstream aerosols in electronic cigarettes, Atmos. Environ., 134, 61–69, https://doi.org/10.1016/j.atmosenv.2016.03.027, 2016.