the Creative Commons Attribution 4.0 License.
the Creative Commons Attribution 4.0 License.
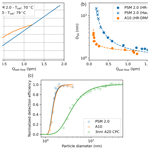
Pushing nano-aerosol measurements towards a new decade – technical note on the Airmodus particle size magnifier 2.0
Joonas Enroth
Aki Pajunoja
Joonas Vanhanen
Katrianne Lehtipalo
Tuukka Petäjä
Markku Kulmala
Accurate measurement of the size distribution of sub-10 nm aerosol particles is still a challenge. Here we introduce a novel version of the Airmodus particle size magnifier (PSM 2.0), which is a condensation-particle-counter-based instrument with a sizing range of 1–12 nm. The extended size range compared to the earlier PSM version enables the direct detection of forming clusters and particles as well as the study of their growth processes without the challenges related to particle charging. It also gives an overlap between the activation size distribution measurements with the PSM and mobility size distribution measurements with conventional mobility particle sizers. We compared the performance of PSM 2.0 to that of a mobility particle size spectrometer, the original A10 particle size magnifier, and a Neutral cluster and Air Ion Spectrometer (NAIS) during field measurements. Also, calibration results were compared against the A10 instrument. The results show that PSM 2.0 is able to activate sub-2 nm clusters and that the concentration and size distribution between 2–12 nm compare well, especially with the NAIS.
- Article
(1141 KB) - Full-text XML
-
Supplement
(657 KB) - BibTeX
- EndNote
Understanding and quantifying the process of new particle formation require direct observations of the forming particles in the relevant size range, no matter the environment. An estimated 40 %–80 % of total aerosol number concentration is formed through new particle formation (Gordon et al., 2017; Dunne et al., 2016; Weagle et al., 2018), even in megacities (Kulmala et al., 2021) Therefore understanding this process is important for reducing the uncertainties in aerosol climate effects as well as health effects of nanoparticles. New particle formation (NPF) is a complicated process which is typically characterized by the formation of clusters in the size range of 1–2 nm that may, under favourable conditions, grow further into nucleation-mode sizes and finally into climate-relevant sizes, potentially acting as cloud condensation nuclei. Current research indicates that the initial cluster formation and growth beyond 2 nm are two separate processes (Tröstl et al., 2016; Kulmala et al., 2004) and can include partly different precursor vapours. The formation and growth of the smallest particles from the sub-3 nm range to 10 nm and beyond are key steps in understanding how many freshly formed particles survive to contribute to the particle loading in the atmosphere. Earlier studies have observed a large number of particles in the sub-3 nm size range, e.g. in fresh traffic emissions (Rönkkö et al., 2017; Lintusaari et al., 2023), in boreal forests (Kulmala et al., 2013; Sulo et al., 2021), in polluted environments (Kontkanen et al., 2017; Zhou et al., 2020; Kulmala et al., 2021), in rainforests (Wimmer et al., 2018), in arctic environments (Sipilä et al., 2021) and on mountaintops (Bianchi et al., 2020) as well as in industry (Ahonen et al., 2017; Sarnela et al., 2015) and indoor air (Farmer et al., 2019).
The capacity to measure and size aerosol particles routinely down to 1 nm in size has only been developed in the past decade, with instruments such as the particle size magnifier (PSM; Vanhanen et al., 2011) and the Neutral cluster and Air Ion Spectrometer (NAIS; Mirme and Mirme, 2013) bridging the gap between mass spectrometers and particle counters. However, measuring the smallest particles and clusters is a challenging task, and each measurement device has its own challenges (Kangasluoma and Kontkanen, 2017). These issues are typically related to the way the instruments make particularly the sub-3 nm particles detectable, through either charging or condensational growth. Electrical mobility spectrometers suffer from low charging probabilities of the smallest particles and the fact that charger ions typically are of similar size to the actual sample in the sub-3 nm range (Kangasluoma et al., 2018), while condensational growth methods must achieve a high enough supersaturation of the working fluid to overcome the Kelvin effect, which typically leads to homogeneous nucleation. However, in practice the condensation particle counter (CPC) techniques utilize both heterogeneous nucleation on insoluble particles and the nano-Köhler process in the case of soluble particles (Kulmala et al., 2007). Currently, observing particle growth from the 1–2 nm size range up to nucleation- and accumulation-mode sizes requires a combination of multiple instruments such as in Kulmala et al. (2013) and Stolzenburg et al. (2022). Although some methods for combining particle size distributions from several different instruments exist and have produced excellent results (Stolzenburg et al., 2022), they still require data overlap between the instruments and assumptions about the instrument detection efficiencies and losses. Moreover, this requires a battery of instruments in order to resolve the size distribution of aerosol particles between 1 and 10 nm. Here we introduce an improved version of the Airmodus A10, called PSM 2.0. The new instrument extends the upper size limit from 4 to 12 nm, without losing accuracy or detection efficiency of the smallest particles.
The aim of this article is to introduce the new PSM 2.0 prototype and its conceptual design, showcase its capacity to measure the size distribution of 1 to 12 nm particles, and compare its performance with the earlier PSM version (A10) and with mobility particle sizers (NAIS and a differential mobility particle sizer, DMPS) during field measurements. A full characterization of the instrument will be the subject of future studies.
Condensation particle counters (CPCs) are commonly used for measuring the total particle number concentration above a certain diameter (cut-off size), which depends mainly on the supersaturation achieved inside the instrument. Aerosol particle sizing with an adjustable supersaturation has been used previously. Related instruments include the original particle size magnifier (Seto et al., 1997), a CPC with variable supersaturation (VSCPS; Gallar et al., 2006) and the original Airmodus PSM (Vanhanen et al., 2011). The required supersaturation for particle activation in a CPC is typically achieved in one of three ways (McMurry, 2000): adiabatic expansion, turbulent mixing or laminar diffusive activation. The design of PSM 2.0 is a hybrid, which uses turbulent mixing to rapidly mix the sample and saturator flows. However, unlike in a mixing-type CPC, the adiabatic mixing does not activate the particles, but in fact the activation is based on a laminar diffusive supersaturation. To further reduce the mixing-type activation inside the mixing section, the inlet of the PSM is slightly heated (40 ∘C). In this, the working principle of PSM 2.0 is similar to that of a recent version of the VSCPS technology by Attoui et al. (2023), with their two-flow mixing system.
The original design of the Airmodus A10 is described in detail by Vanhanen et al. (2011). In that instrument, size-selective particle activation and growth were achieved by varying the saturator flow rate between 0.1 and 1.3 L min−1. The inlet flow rate of the instrument was held constant by changing the excess flow rate after the condenser. This varying saturator flow led to a varying total flow rate through the most crucial part of the instrument – the mixing section. With the varying flow rate, the temperature and the vapour amount also varied, causing the instrument to find a dynamic equilibrium, which can be susceptible to instability.
PSM 2.0, in contrast, has a constant flow rate passing through the mixing section in addition to constant, and temperature-controlled, inlet flow. The only parameter which is varied is the ratio of the vapour amount arriving at the mixing section from the saturator. The total flow containing both dry and wet flow is referred to as the saturator flow. Similarly to the instrument of Attoui et al. (2023), two mass flow controllers are operated in tandem to produce a constant 2 L min−1 flow rate. One mass flow controller regulates the amount of dry air reaching the heater prior to the mixing section, while the other one controls the flow passing through the saturator prior to entering the same heater element as the dry flow (Fig. 1). This means that although the saturator flow rate is defined similarly to in the A10, the flow into the mixing piece is kept constant by the addition of a heated dry flow, thus allowing the supersaturation of the instrument to be varied without changing the flow rate into the mixing piece.
In the prototype PSM 2.0, the total saturator flow rate has been increased to 2 L min−1, of which at most 1.9 L min−1 can be wet saturator flow. Also, the inlet flow rate has been decreased to 1.5 L min−1. This means that the highest wet saturator flow rate in the instrument has increased almost 60 % compared to the A10, allowing us to reach the same maximum supersaturation with a saturator temperature up to 10 ∘C lower, as shown in Fig. 2a. The difference is only partially explained by the difference in flows and to a large degree by a redesigned saturator. Unlike in the A10, the wet flow is fully saturated even at the highest flow rates, which enables the lowering of the saturator temperature without compromising on the resulting vapour pressure and hence the maximum achieved supersaturation. The lower temperature of the saturator is key for enabling the extended size range of PSM 2.0 (Fig. 2b). It means that the total vapour pressure of diethylene glycol (DEG) after the mixing section is more than 5 times lower than it is in the A10, allowing for much finer control of the supersaturation and therefore particle activation and growth. The duration of a single scan (240 s) has remained the same as in the A10, but the increased stability allows for increasing the time resolution by analysing the upward and downward scans separately unlike with the A10. A potential further increase in the time resolution is a subject for future characterization studies. D50 as a function of the wet flow behaves exponentially due to the Kelvin effect, but in PSM 2.0 the behaviour is clearly more linear compared to the A10 even though the sizing capability is increased from 1–4 to 1–12 nm. D50 is the particle diameter at which 50 % of the particles are activated at a given flow rate. As shown in Vanhanen et al. (2011) and elsewhere, the particle activation in the A10 is not fully consistent with ideal mixing-type activation. The design of PSM 2.0 was intentionally moved further away from the mixing-type activation and towards diffusive activation. The temperature of the heater is held intentionally at a higher temperature than the saturator. This not only prevents the condensation of DEG into the heater, but also ensures that, even in the case of adiabatic mixing, the temperature after mixing is sufficiently high to keep the saturation ratio below unity. The aerosol carrier flow is still turbulently mixed with the saturator flow as in the A10, but here the turbulent mixing is only a method to achieve a uniform vapour pressure across the aerosol sample flow efficiently and rapidly. This also reduces the diffusional losses of sample compared to more laminar designs.
The size-selective particle activation is dependent on the homogeneity of the supersaturation field across the aerosol sample and is reflected, for example, in the steepness of the cut-off curve. As shown by previous work, there are two commonly used approaches in continuous-flow instruments to achieve a sharp cut-off curve. One is to use adiabatic expansion, but this results in only semi-continuous sampling. The second and more common solution is to use a core-fed aerosol flow together with a vapour sheath, which will result in the aerosol experiencing a more homogeneous supersaturation field across its streamlines. Here, we use the opposite approach to this method. The design of the PSM condenser is such that it effectively performs core sampling of the saturated flow mixed with the aerosol. While this design “wastes” both aerosol sample and DEG vapour, it is easy to control and results in an equally sharp cut-off curve. Hence, we can achieve a sharper cut-off response than in a single-flow instrument (Fig. 2c, which is required for the extended size range). A regular CPC is used for subsequent particle detection. In this study, the CPC used was an Airmodus A20.
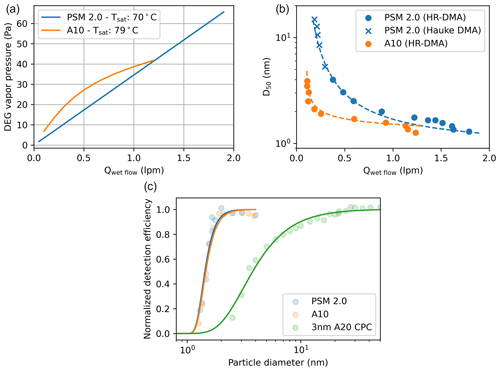
Figure 2(a) Modelled DEG vapour pressure at the mixing section of the A10 and PSM 2.0. (b) Measured calibration curve of the PSM 2.0 prototype and an original A10. The increased linearity in the vapour pressure, as a function of the saturator flow, enables PSM 2.0 to have a larger activation diameter range than the A10. D50 is the particle diameter at which 50 % of the particles are activated at a given flow rate. (c) Cut-off curves for the highest saturator flow for the A10 and prototype PSM 2.0 and a 3 nm cut-off version of the full-flow A20 CPC, showing the considerable steeper cut-off curves achieved with the PSM technology.
The cut-off curve (detection efficiency as a function of size) for PSM 2.0 compared to the A10 PSM and A20 CPC is shown in Fig. 2c. The results show that the lowest cut-off size of PSM 2.0 is comparable to the A10 PSM, and for both of them the cut-off size is much lower than for the A20 CPC with a nominal 3 nm cut-off size. The setup used for the A10 and PSM 2.0 calibration consisted of two distinct configurations. For the sub-5 nm particles, we used the NiCr particles, size-selected with a half-mini differential mobility analyser (Fernández de la Mora and Kozlowski, 2013) that was calibrated with tetraheptylammonium bromide mobility standards. As our setup did not permit particles larger than 4.5 nm to be selected with this setup, we used a Hauke-type differential mobility analyser (e.g. Winklmayr et al., 1991) to produce monodisperse silver particles of up to 12 nm.
To test the performance of the prototype PSM 2.0 in field conditions and to compare it to other instruments measuring the particle number concentration and size distribution, we performed side-by-side measurements at the SMEAR III station in Helsinki during May 2023. SMEAR III is an urban background station located in the Kumpula Campus area ca. 4 km from the Helsinki city centre (Järvi et al., 2009). The inversion method used to resolve aerosol particle number size distributions was the stepwise method (Lehtipalo et al., 2014). The inversion methods used for the A10 are not yet fully optimized for PSM 2.0, and full development of the inversion methods, including kernel and EM methods, is a subject for further study. Initial inversion results were able to resolve 12 size bins in the 1–12 nm size range.
First, we investigated how well PSM 2.0 is able to detect the smallest atmospheric particles and ions. In Fig. 3 we show the total concentration of sub-2 nm particles measured by PSM 2.0 as well as its predecessor, the A10, and compare those to the concentration of sub-2 nm air ions (sum of positive + negative cluster ions) measured by the Neutral cluster and Air Ion Spectrometer (NAIS; Mirme and Mirme, 2013). We observe that during the nighttime, PSM 2.0 measured concentrations similar to the ion concentrations measured with the NAIS, showing that it is able to activate most of the atmospheric cluster ions. During daylight (the sunrise in Helsinki in May is at around 04:00 and sunset is at 20:30 local winter time), the concentration measured by PSM 2.0 was higher than the cluster ion concentration, probably due to neutral clustering of low-volatility vapours that form due to photochemical reactions (Jokinen et al., 2017), although contribution from traffic cannot be excluded. We were also able to capture a new-particle-formation event around midday, characterized by a sudden clear increase in the sub-2 nm particles and showcasing the instrument's sensitivity in both large and small concentration ranges. The concentration measured by the A10 PSM was clearly lower than that measured by PSM 2.0, probably due to limitations in the activation efficiency of the smallest particles. It has also been previously seen that the A10 PSM does not detect all of the atmospheric sub-2 nm ions, although it is sensitive to sub-3 nm particles formed especially during new particle formation (Sulo et al., 2021), as also seen in Fig. 3. Uncertainties in Fig. 3 and subsequent figures were calculated by determining the systematic uncertainties for each instrument such as the amplitude of random fluctuations in CPCs and uncertainty in electrometer currents and including random uncertainty in charging and sizing using a Monte Carlo simulation similarly to Kangasluoma et al. (2020).
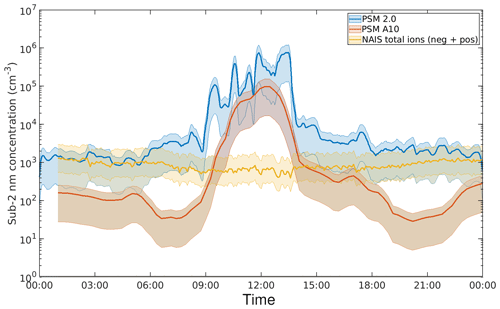
Figure 3Cluster concentrations in the sub-2 nm size range observed with PSM 2.0 and the A10 PSM in blue and orange, respectively, and total sub-2 nm ion concentrations measured by the NAIS in yellow on 9 May 2023. The concentration uncertainties were calculated in the same way as in Kangasluoma et al. (2020). The time is local time.
The 2–7 nm size range is often considered the key size range for new particle formation (Leino et al., 2016; Tammet et al., 2014) and in particular the key size range for determining whether freshly formed particles grow into climate-relevant sizes. Our results (Figs. 4a, S1) show that the prototype PSM 2.0 measured very similar total concentration in the 2.5–7 nm size range to the NAIS in particle mode, without the need for charging the sample. In the 7–12 nm size range (Figs. 4b, S2), PSM 2.0 agreed rather well with the differential mobility particle sizer (DMPS). The NAIS consistently saw somewhat higher concentrations than the DMPS and PSM 2.0. This feature has been seen in many previous instrument comparisons (Gagné et al., 2011; Kangasluoma et al., 2020), and more sophisticated DMPS systems tuned to measure ultrafine particles (e.g. those used in Kangasluoma et al., 2020) could provide even better agreement with PSM 2.0. The differences in the DMPS- and PSM 2.0-measured concentrations are likely due to reduced counting statistics in the DMPS (Stolzenburg et al., 2023). During the new-particle-formation event with higher concentrations in this size range (ca. 12–14 pm), all of the instruments agreed well.
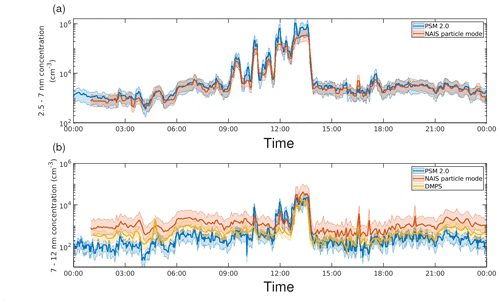
Figure 4(a) The concentration of 2.5–7 nm aerosols measured by PSM 2.0 and the NAIS in particle mode. (b) The concentration of 7–12 nm concentrations measured with PSM 2.0, the NAIS in particle mode and the DMPS. The concentration uncertainties were calculated in the same way as in Kangasluoma et al. (2020). The time is local time.
Finally, we investigated the whole size distribution of 1–12 nm particles during and outside the new-particle-formation event time (Fig. 5). Our measurements show a rather good agreement between PSM 2.0 and the size distributions measured by the other instruments, especially considering that the PSM determines particle size based on a totally different principle (particle activation) than the mobility particle sizers. The most notable differences in the size distribution are seen in the 2–4 nm size range. The 2–4 nm size distribution is known to be very difficult to measure accurately, as most instruments suffer from significant losses and/or low charging probabilities and because solving the size distribution in this range includes large uncertainties (Kangasluoma et al., 2016, 2020). Figure 5 also shows that although the total particle number concentration in the 2.5–7 nm size range is very similar between the NAIS and PSM 2.0, there exist differences between the number concentrations of different size particles within that size range as measured by the NAIS or PSM 2.0. The drop in the NAIS particle-mode concentration close to 2–3 nm could also be related to the instrument post-filter designed to remove ions formed in the corona charger (Manninen et al., 2016), and a similar cross-over (i.e. the NAIS showing more than other instruments above 3 nm but less between 2–3 nm) has also been seen in earlier instrument comparisons (Kangasluoma et al., 2020). The DMPS sees clearly lower concentrations than the other instruments below ca. 5 nm during the event and below ca. 8 nm during night. Overall, it is likely that the improved stability and sensitivity of PSM 2.0 allow us to more accurately detect the particle size distribution below 4 nm than previously.
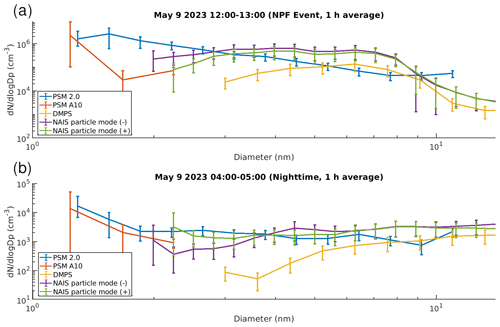
Figure 5The size distribution measured with PSM 2.0, the A10 PSM, the DMPS, and the NAIS in particle mode in negative and positive polarizations during an NPF event (a) and during the nighttime (b). The concentration uncertainties were calculated in the same way as in Kangasluoma et al. (2020). The time is local time.
Here we presented a new prototype version of the Airmodus particle size magnifier, namely PSM 2.0. The new instrument is capable of detecting sub-2 nm clusters, intermediate particles (2–7 nm) and also the lower end of the nucleation mode (7–12 nm). It is significantly more effective in activating clusters in the sub-2 nm size range than the previous PSM versions; e.g. it is able to observe in practice all small ions during the nighttime and also enhanced concentrations of sub-2 nm particles during NPF events, and therefore it improves the closure between predicted and observed cluster concentrations (Kulmala et al., 2022). Furthermore, the number concentrations of 2–7 nm particles measured by PSM 2.0 and the NAIS agreed very well, although the detailed size distributions show some differences between different instrument types. These results are consistent with earlier instrument comparisons (Kangasluoma et al., 2020; Stolzenburg et al., 2022), showing the need for instrumentation optimized for the sub-10 nm size range.
Additionally, PSM 2.0 should be significantly more stable than the PSM A10 due to the redesign of the saturator flow system, flow mixing and internal liquid handling, which allows for finer control of the supersaturation and reduces uncertainty from turbulent mixing and non-uniform supersaturation. This improves its applicability in field conditions. Proper characterization of the instrument stability is a subject for future work. This work should include studies about the sizing accuracy; seed material dependency, especially at the larger sizes; and the time resolution limits of PSM 2.0. It is currently the only instrument capable of measuring the whole size range relevant for particle formation and early growth without needing to charge particles. Therefore, we can state that PSM 2.0 could become revolutionary in the investigation of the dynamics of sub-10 nm clusters and particles.
The data used are available at https://doi.org/10.5281/zenodo.10663799 (Sulo, 2024). The data are licensed under a Creative Commons 4.0 Attribution (CC BY) licence.
The supplement related to this article is available online at: https://doi.org/10.5194/ar-2-13-2024-supplement.
JE and JV designed the instrument. JE, JV and AP participated in the laboratory calibration. JE performed the modelling. JS and AP participated in the field measurements. JS, JE and JV performed the data analysis, while JS, JE, JV, KL and MK wrote the paper. TP and MK facilitated access to research infrastructure. All authors contributed comments to the article.
At least one of the (co-)authors is a member of the editorial board of Aerosol Research. The peer-review process was guided by an independent editor. Katrianne Lehtipalo, Markku Kulmala, Joonas Enroth, Joonas Vanhanen and Aki Pajunoja are shareholders of Airmodus Oy. Joonas Enroth, Joonas Vanhanen and Aki Pajunoja work for Airmodus Oy.
Publisher's note: Copernicus Publications remains neutral with regard to jurisdictional claims made in the text, published maps, institutional affiliations, or any other geographical representation in this paper. While Copernicus Publications makes every effort to include appropriate place names, the final responsibility lies with the authors.
We acknowledge the following projects: ACCC Flagship funded by the Research Council of Finland, grant numbers 337549 (UH) and 337552 (FMI); an academy professorship funded by the Research Council of Finland (grant no. 302958); Research Council of Finland projects nos. 1325656, 311932, 334792, 316114, 325647, 325681 and 347782; the “Quantifying carbon sink, CarbonSink+ and their interaction with air quality” INAR project funded by the Jane and Aatos Erkko Foundation; the “Gigacity” project funded by the Wihuri Foundation and the European Research Council (ERC) project ATM-GTP, contract no. 742206. University of Helsinki support via ACTRIS-HY is acknowledged. Support from the technical and scientific staff in Kumpula is acknowledged.
This research has been supported by the Research Council of Finland (grant nos. 337549, 337552, 302958, 1325656, 311932, 334792, 316114, 325647, 325681, and 347782); the Jane ja Aatos Erkon Säätiö (project CarbonSink+); the Wihuri Foundation (project Gigacity); and the European Research Council, H2020 (grant no. 742206).
This paper was edited by Andreas Held and reviewed by two anonymous referees.
Ahonen, L. R., Kangasluoma, J., Lammi, J., Lehtipalo, K., Hämeri, K., Petäjä, T., and Kulmala, M.: First measurements of the number size distribution of 1–2 nm aerosol particles released from Manufacturing Processes in a cleanroom environment, Aerosol Sci. Technol., 51, 685–693, https://doi.org/10.1080/02786826.2017.1292347, 2017.
Attoui, M., Perez-Lorenzo, L. J., Brock, C. A., and Fernandez de la Mora, J.: High resolution characterization of a sheathed axisymmetric variable supersaturation condensation particle sizer, J. Aerosol Sci., 169, 106112, https://doi.org/10.1016/j.jaerosci.2022.106112, 2023.
Bianchi, F., Junninen, H., Bigi, A., Sinclair, V. A., Dada, L., Hoyle, C. R., Zha, Q., Yao, L., Ahonen, L. R., Bonasoni, P., Buenrostro Mazon, S., Hutterli, M., Laj, P., Lehtipalo, K., Kangasluoma, J., Kerminen, V.-M., Kontkanen, J., Marinoni, A., Mirme, S., Molteni, U., Petäjä, T., Riva, M., Rose, C., Sellegri, K., Yan, C., Worsnop, D. R., Kulmala, M., Baltensperger, U., and Dommen, J.: Biogenic particles formed in the Himalaya as an important source of free tropospheric aerosols, Nat. Geosci., 14, 4–9, https://doi.org/10.1038/s41561-020-00661-5, 2020.
Dunne, E. M., Gordon, H., Kürten, A., Almeida, J., Duplissy, J., Williamson, C., Ortega, I. K., Pringle, K. J., Adamov, A., Baltensperger, U., Barmet, P., Benduhn, F., Bianchi, F., Breitenlechner, M., Clarke, A., Curtius, J., Dommen, J., Donahue, N. M., Ehrhart, S., Flagan, R. C., Franchin, A., Guida, R., Hakala, J., Hansel, A., Heinritzi, M., Jokinen, T., Kangasluoma, J., Kirkby, J., Kulmala, M., Kupc, A., Lawler, M. J., Lehtipalo, K., Makhmutov, V., Mann, G., Mathot, S., Merikanto, J., Miettinen, P., Nenes, A., Onnela, A., Rap, A., Reddington, C. L., Riccobono, F., Richards, N. A., Rissanen, M. P., Rondo, L., Sarnela, N., Schobesberger, S., Sengupta, K., Simon, M., Sipilä, M., Smith, J. N., Stozkhov, Y., Tomé, A., Tröstl, J., Wagner, P. E., Wimmer, D., Winkler, P. M., Worsnop, D. R., and Carslaw, K. S.: Global Atmospheric Particle Formation from CERN cloud measurements, Science, 354, 1119–1124, https://doi.org/10.1126/science.aaf2649, 2016.
Fernández de la Mora, J. and Kozlowski, J.: Hand-held differential mobility analyzers of high resolution for 1–30 nm particles: design and fabrication considerations, J. Aerosol. Sci., 57, 45–53, https://doi.org/10.1016/j.jaerosci.2012.10.009, 2013.
Farmer, D. K., Vance, M. E., Abbatt, J. P., Abeleira, A., Alves, M. R., Arata, C., Boedicker, E., Bourne, S., Cardoso-Saldaña, F., Corsi, R., DeCarlo, P. F., Goldstein, A. H., Grassian, V. H., Hildebrandt Ruiz, L., Jimenez, J. L., Kahan, T. F., Katz, E. F., Mattila, J. M., Nazaroff, W. W., Novoselac, A., O'Brien, R. E., Or, V. W., Patel, S., Sankhyan, S., Stevens, P. S., Tian, Y., Wade, M., Wang, C., Zhou, S., and Zhou, Y.: Overview of homechem: House observations of Microbial and Environmental Chemistry, Environ. Sci.-Proc. Imp., 21, 1280–1300, https://doi.org/10.1039/c9em00228f, 2019.
Gagné, S., Lehtipalo, K., Manninen, H. E., Nieminen, T., Schobesberger, S., Franchin, A., Yli-Juuti, T., Boulon, J., Sonntag, A., Mirme, S., Mirme, A., Hõrrak, U., Petäjä, T., Asmi, E., and Kulmala, M.: Intercomparison of air ion spectrometers: an evaluation of results in varying conditions, Atmos. Meas. Tech., 4, 805–822, https://doi.org/10.5194/amt-4-805-2011, 2011.
Gallar, C., Brock, C. A., Jimenez, J. L., and Simons, C.: A variable supersaturation condensation particle sizer, Aerosol Sci. Technol., 40, 431–436, https://doi.org/10.1080/02786820600643339, 2006.
Gordon, H., Kirkby, J., Baltensperger, U., Bianchi, F., Breitenlechner, M., Curtius, J., Dias, A., Dommen, J., Donahue, N. M., Dunne, E. M., Duplissy, J., Ehrhart, S., Flagan, R. C., Frege, C., Fuchs, C., Hansel, A., Hoyle, C. R., Kulmala, M., Kürten, A., Lehtipalo, K., Makhmutov, V., Molteni, U., Rissanen, M. P., Stozkhov, Y., Tröstl, J., Tsagkogeorgas, G., Wagner, R., Williamson, C., Wimmer, D., Winkler, P. M., Yan, C. and Carslaw, K. S.: Causes and importance of new particle formation in the present‐day and preindustrial atmospheres, J. Geophys. Res.-Atmos., 122, 8739–8760, https://doi.org/10.1002/2017jd026844, 2017.
Järvi, L., Hannuniemi, H., Hussein, T., Junninen, H., Aalto, P. P., Hillamo, R., Mäkelä, T., Keronen, P., Siivola, E., Vesala, T., and Kulmala, M.: The urban measurement station SMEAR III: Continuous monitoring of air pollution and surface-atmosphere interactions in Helsinki, Finland, Boreal Environ. Res., 14, 86–109, 2009.
Jokinen, T., Kontkanen, J., Lehtipalo, K., Manninen, H. E., Aalto, J., Porcar-Castell, A., Garmash, O., Nieminen, T., Ehn, M., Kangasluoma, J., Junninen, H., Levula, J., Duplissy, J., Ahonen, L. R., Rantala, P., Heikkinen, L., Yan, C., Sipilä, M., Worsnop, D. R., Bäck, J., Petäjä, T., Kerminen, V.-M., and Kulmala, M.: Solar eclipse demonstrating the importance of photochemistry in New Particle Formation, Sci. Rep., 7, 45707, https://doi.org/10.1038/srep45707, 2017.
Kangasluoma, J. and Kontkanen, J.: On the sources of uncertainty in the sub-3 nm particle concentration measurement, J. Aerosol Sci., 112, 34–51, https://doi.org/10.1016/j.jaerosci.2017.07.002, 2017.
Kangasluoma, J., Franchin, A., Duplissy, J., Ahonen, L., Korhonen, F., Attoui, M., Mikkilä, J., Lehtipalo, K., Vanhanen, J., Kulmala, M., and Petäjä, T.: Operation of the Airmodus A11 nano Condensation Nucleus Counter at various inlet pressures and various operation temperatures, and design of a new inlet system, Atmos. Meas. Tech., 9, 2977–2988, https://doi.org/10.5194/amt-9-2977-2016, 2016.
Kangasluoma, J., Ahonen, L. R., Laurila, T. M., Cai, R., Enroth, J., Mazon, S. B., Korhonen, F., Aalto, P. P., Kulmala, M., Attoui, M., and Petäjä, T.: Laboratory verification of a new high flow differential mobility particle sizer, and field measurements in Hyytiälä, J. Aerosol Sci., 124, 1–9, https://doi.org/10.1016/j.jaerosci.2018.06.009, 2018.
Kangasluoma, J., Cai, R., Jiang, J., Deng, C., Stolzenburg, D., Ahonen, L. R., Chan, T., Fu, Y., Kim, C., Laurila, T. M., Zhou, Y., Dada, L., Sulo, J., Flagan, R. C., Kulmala, M., Petäjä, T., and Lehtipalo, K.: Overview of measurements and current instrumentation for 1–10 nm aerosol particle number size distributions, J. Aerosol Sci., 148, 105584, https://doi.org/10.1016/j.jaerosci.2020.105584, 2020.
Kontkanen, J., Lehtipalo, K., Ahonen, L., Kangasluoma, J., Manninen, H. E., Hakala, J., Rose, C., Sellegri, K., Xiao, S., Wang, L., Qi, X., Nie, W., Ding, A., Yu, H., Lee, S., Kerminen, V.-M., Petäjä, T., and Kulmala, M.: Measurements of sub-3 nm particles using a particle size magnifier in different environments: from clean mountain top to polluted megacities, Atmos. Chem. Phys., 17, 2163–2187, https://doi.org/10.5194/acp-17-2163-2017, 2017.
Kulmala, M., Vehkamäki, H., Petäjä, T., Dal Maso, M., Lauri, A., Kerminen, V.-M., Birmili, W., and McMurry, P. H.: Formation and growth rates of ultrafine atmospheric particles: A review of observations, J. Aerosol Sci., 35, 143–176, https://doi.org/10.1016/j.jaerosci.2003.10.003, 2004.
Kulmala, M., Mordas, G., Petäjä, T., Grönholm, T., Aalto, P. P., Vehkamäki, H., Hienola, A. I., Herrmann, E., Sipilä, M., Riipinen, I., Manninen, H. E., Hämeri, K., Stratmann, F., Bilde, M., Winkler, P. M., Birmili, W., and Wagner, P. E.: The Condensation Particle Counter Battery (CPCB): A new tool to investigate the activation properties of nanoparticles, J. Aerosol Sci., 38, 289–304, https://doi.org/10.1016/j.jaerosci.2006.11.008, 2007.
Kulmala, M., Kontkanen, J., Junninen, H., Lehtipalo, K., Manninen, H. E., Nieminen, T., Petäjä, T., Sipilä, M., Schobesberger, S., Rantala, P., Franchin, A., Jokinen, T., Järvinen, E., Äijälä, M., Kangasluoma, J., Hakala, J., Aalto, P. P., Paasonen, P., Mikkilä, J., Vanhanen, J., Aalto, J., Hakola, H., Makkonen, U., Ruuskanen, T., Mauldin, R. L., Duplissy, J., Vehkamäki, H., Bäck, J., Kortelainen, A., Riipinen, I., Kurtén, T., Johnston, M. V., Smith, J. N., Ehn, M., Mentel, T. F., Lehtinen, K. E., Laaksonen, A., Kerminen, V.-M., and Worsnop, D. R.: Direct observations of atmospheric aerosol nucleation, Science, 339, 943–946, https://doi.org/10.1126/science.1227385, 2013.
Kulmala, M., Dada, L., Daellenbach, K. R., Yan, C., Stolzenburg, D., Kontkanen, J., Ezhova, E., Hakala, S., Tuovinen, S., Kokkonen, T. V., Kurppa, M., Cai, R., Zhou, Y., Yin, R., Baalbaki, R., Chan, T., Chu, B., Deng, C., Fu, Y., Ge, M., He, H., Heikkinen, L., Junninen, H., Liu, Y., Lu, Y., Nie, W., Rusanen, A., Vakkari, V., Wang, Y., Yang, G., Yao, L., Zheng, J., Kujansuu, J., Kangasluoma, J., Petäjä, T., Paasonen, P., Järvi, L., Worsnop, D., Ding, A., Liu, Y., Wang, L., Jiang, J., Bianchi, F. and Kerminen, V.-M.: Is reducing new particle formation a plausible solution to mitigate particulate air pollution in Beijing and other Chinese megacities?, Faraday Discuss., 226, 334–347, https://doi.org/10.1039/d0fd00078g, 2021.
Kulmala, M., Stolzenburg, D., Dada, L., Cai, R., Kontkanen, J., Yan, C., Kangasluoma, J., Ahonen, L. R., Gonzalez-Carracedo, L., Sulo, J., Tuovinen, S., Deng, C., Li, Y., Lehtipalo, K., Lehtinen, K. E. J., Petäjä, T., Winkler, P. M., Jiang, J., and Kerminen, V.-M.: Towards a concentration closure of sub-6 nm aerosol particles and sub-3 nm atmospheric clusters, J. Aerosol Sci., 159, 105878, https://doi.org/10.1016/j.jaerosci.2021.105878, 2022.
Lehtipalo, K., Leppä, J., Kontkanen, J., Kangasluoma, J., Franchin, A.,Wimmer, D., Schobesberger, S., Junninen, H., Petäjä, T., Sipilä, M., Mikkilä, J., Vanhanen, J., Worsnop, D. R., and Kulmala, M.: Methods for determining particle size distribution and growth rates between 1–3 nm using the Particle Size Magnifier, Boreal Environ. Res., 19, 215–236, 2014.
Leino, K., Nieminen, T., Manninen, H., Petäjä, T., Kerminen, V.-M., and Kulmala, M.: Intermediate ions as a strong indicator of new particle formation bursts in boreal forest, Boreal Environ. Res., 21, 274–286, 2016.
Lintusaari, H., Kuuluvainen, H., Vanhanen, J., Salo, L., Portin, H., Järvinen, A., Juuti, P., Hietikko, R., Teinilä, K., Timonen, H., Niemi, J. V., and Rönkkö, T.: Sub-23 nm particles dominate non-volatile particle number emissions of road traffic, Environ. Sci. Technol., 57, 10763–10772, https://doi.org/10.1021/acs.est.3c03221, 2023.
Manninen, H. E., Mirme, S., Mirme, A., Petäjä, T., and Kulmala, M.: How to reliably detect molecular clusters and nucleation mode particles with Neutral cluster and Air Ion Spectrometer (NAIS), Atmos. Meas. Tech., 9, 3577–3605, https://doi.org/10.5194/amt-9-3577-2016, 2016.
McMurry, P.: A review of atmospheric aerosol measurements, Atmos. Environ., 34, 1959–1999, https://doi.org/10.1016/s1352-2310(99)00455-0, 2000.
Mirme, S. and Mirme, A.: The mathematical principles and design of the NAIS – a spectrometer for the measurement of cluster ion and nanometer aerosol size distributions, Atmos. Meas. Tech., 6, 1061–1071, https://doi.org/10.5194/amt-6-1061-2013, 2013.
Rönkkö, T., Kuuluvainen, H., Karjalainen, P., Keskinen, J., Hillamo, R., Niemi, J. V., Pirjola, L., Timonen, H. J., Saarikoski, S., Saukko, E., Järvinen, A., Silvennoinen, H., Rostedt, A., Olin, M., Yli-Ojanperä, J., Nousiainen, P., Kousa, A., and Dal Maso, M.: Traffic is a major source of atmospheric nanocluster aerosol, P. Natl. Acad. Sci. USA, 114, 7549–7554, https://doi.org/10.1073/pnas.1700830114, 2017.
Sarnela, N., Jokinen, T., Nieminen, T., Lehtipalo, K., Junninen, H., Kangasluoma, J., Hakala, J., Taipale, R., Schobesberger, S., Sipilä, M., Larnimaa, K., Westerholm, H., Heijari, J., Kerminen, V.-M., Petäjä, T., and Kulmala, M.: Sulphuric acid and aerosol particle production in the vicinity of an oil refinery, Atmos. Environ., 119, 156–166, https://doi.org/10.1016/j.atmosenv.2015.08.033, 2015.
Seto, T., Nakamoto, T., Okuyama, K., Adachi, M., Kuga, Y., and Takeuchi, K.: Size distribution measurement of nanometer-sized aerosol particles using DMA under low-pressure conditions, J. Aerosol Sci., 28, 193–206, https://doi.org/10.1016/s0021-8502(96)00071-7, 1997.
Sipilä, M., Sarnela, N., Neitola, K., Laitinen, T., Kemppainen, D., Beck, L., Duplissy, E.-M., Kuittinen, S., Lehmusjärvi, T., Lampilahti, J., Kerminen, V.-M., Lehtipalo, K., Aalto, P. P., Keronen, P., Siivola, E., Rantala, P. A., Worsnop, D. R., Kulmala, M., Jokinen, T., and Petäjä, T.: Wintertime subarctic new particle formation from Kola Peninsula sulfur emissions, Atmos. Chem. Phys., 21, 17559–17576, https://doi.org/10.5194/acp-21-17559-2021, 2021.
Stolzenburg, D., Ozon, M., Kulmala, M., Lehtinen, K. E. J., Lehtipalo, K., and Kangasluoma, J.: Combining instrument inversions for sub-10 nm aerosol number size-distribution measurements, J. Aerosol Sci., 159, 105862, https://doi.org/10.1016/j.jaerosci.2021.105862, 2022.
Stolzenburg, D., Laurila, T., Aalto, P., Vanhanen, J., Petäjä, T., and Kangasluoma, J.: Improved counting statistics of an ultrafine differential mobility particle size spectrometer system, Atmos. Meas. Tech., 16, 2471–2483, https://doi.org/10.5194/amt-16-2471-2023, 2023.
Sulo, J.: Dataset for: Pushing nano-aerosol measurements towards a new decade – Technical note on Airmodus Particle Size Magnifier 2.0, Zenodo [data set], https://doi.org/10.5281/zenodo.10663800, 2024.
Sulo, J., Sarnela, N., Kontkanen, J., Ahonen, L., Paasonen, P., Laurila, T., Jokinen, T., Kangasluoma, J., Junninen, H., Sipilä, M., Petäjä, T., Kulmala, M., and Lehtipalo, K.: Long-term measurement of sub-3 nm particles and their precursor gases in the boreal forest, Atmos. Chem. Phys., 21, 695–715, https://doi.org/10.5194/acp-21-695-2021, 2021.
Tammet, H., Komsaare, K., and Hõrrak, U.: Intermediate ions in the atmosphere, Atmos. Res., 135–136, 263–273, https://doi.org/10.1016/j.atmosres.2012.09.009, 2014.
Tröstl, J., Chuang, W. K., Gordon, H., Heinritzi, M., Yan, C., Molteni, U., Ahlm, L., Frege, C., Bianchi, F., Wagner, R., Simon, M., Lehtipalo, K., Williamson, C., Craven, J. S., Duplissy, J., Adamov, A., Almeida, J., Bernhammer, A.-K., Breitenlechner, M., Brilke, S., Dias, A., Ehrhart, S., Flagan, R. C., Franchin, A., Fuchs, C., Guida, R., Gysel, M., Hansel, A., Hoyle, C. R., Jokinen, T., Junninen, H., Kangasluoma, J., Keskinen, H., Kim, J., Krapf, M., Kürten, A., Laaksonen, A., Lawler, M., Leiminger, M., Mathot, S., Möhler, O., Nieminen, T., Onnela, A., Petäjä, T., Piel, F. M., Miettinen, P., Rissanen, M. P., Rondo, L., Sarnela, N., Schobesberger, S., Sengupta, K., Sipilä, M., Smith, J. N., Steiner, G., Tomè, A., Virtanen, A., Wagner, A. C., Weingartner, E., Wimmer, D., Winkler, P. M., Ye, P., Carslaw, K. S., Curtius, J., Dommen, J., Kirkby, J., Kulmala, M., Riipinen, I., Worsnop, D. R., Donahue, N. M., and Baltensperger, U.: The role of low-volatility organic compounds in initial particle growth in the atmosphere, Nature, 533, 527–531, https://doi.org/10.1038/nature18271, 2016.
Vanhanen, J., Mikkilä, J., Lehtipalo, K., Sipilä, M., Manninen, H. E., Siivola, E., Petäjä, T., and Kulmala, M.: Particle size magnifier for nano-CN detection, Aerosol Sci. Technol., 45, 533–542, https://doi.org/10.1080/02786826.2010.547889, 2011.
Weagle, C. L., Snider, G., Li, C., van Donkelaar, A., Philip, S., Bissonnette, P., Burke, J., Jackson, J., Latimer, R., Stone, E., Abboud, I., Akoshile, C., Anh, N. X., Brook, J. R., Cohen, A., Dong, J., Gibson, M. D., Griffith, D., He, K. B., Holben, B. N., Kahn, R., Keller, C. A., Kim, J. S., Lagrosas, N., Lestari, P., Khian, Y. L., Liu, Y., Marais, E. A., Martins, J. V., Misra, A., Muliane, U., Pratiwi, R., Quel, E. J., Salam, A., Segev, L., Tripathi, S. N., Wang, C., Zhang, Q., Brauer, M., Rudich, Y., and Martin, R. V.: Global Sources of Fine Particulate matter: Interpretation of PM2.5 chemical composition observed by Spartan using a global chemical transport model, Environ. Sci. Technol., 52, 11670–11681, https://doi.org/10.1021/acs.est.8b01658, 2018.
Wimmer, D., Buenrostro Mazon, S., Manninen, H. E., Kangasluoma, J., Franchin, A., Nieminen, T., Backman, J., Wang, J., Kuang, C., Krejci, R., Brito, J., Goncalves Morais, F., Martin, S. T., Artaxo, P., Kulmala, M., Kerminen, V.-M., and Petäjä, T.: Ground-based observation of clusters and nucleation-mode particles in the Amazon, Atmos. Chem. Phys., 18, 13245–13264, https://doi.org/10.5194/acp-18-13245-2018, 2018.
Winklmayr, W., Reischl, G. P., Lindner, A. O., and Berner, A.: A New Electromobility Spectrometer for the Measurement of Aerosol Size Distributions in the Size Range from 1 to 1000 Nm, J. Aerosol. Sci., 22, 289–296, https://doi.org/10.1016/S0021-8502(05)80007-2, 1991.
Zhou, Y., Dada, L., Liu, Y., Fu, Y., Kangasluoma, J., Chan, T., Yan, C., Chu, B., Daellenbach, K. R., Bianchi, F., Kokkonen, T. V., Liu, Y., Kujansuu, J., Kerminen, V.-M., Petäjä, T., Wang, L., Jiang, J., and Kulmala, M.: Variation of size-segregated particle number concentrations in wintertime Beijing, Atmos. Chem. Phys., 20, 1201–1216, https://doi.org/10.5194/acp-20-1201-2020, 2020.
We present a novel version of an aerosol number size distribution instrument, showcasing its capability to measure particle number concentration and particle number size distribution between 1 and 12 nm. Our results show that the instrument agrees well with existing instrumentation and allows for both the accurate measurement of the smallest particles and overlap with more conventional aerosol number size distribution instruments.
We present a novel version of an aerosol number size distribution instrument, showcasing its...