the Creative Commons Attribution 4.0 License.
the Creative Commons Attribution 4.0 License.
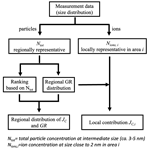
Opinion: A paradigm shift in investigating the general characteristics of atmospheric new particle formation using field observations
Markku Kulmala
Diego Aliaga
Santeri Tuovinen
Runlong Cai
Heikki Junninen
Federico Bianchi
Yafang Cheng
Aijun Ding
Douglas R. Worsnop
Tuukka Petäjä
Katrianne Lehtipalo
Pauli Paasonen
Veli-Matti Kerminen
Atmospheric new particle formation (NPF) and associated production of secondary particulate matter dominate aerosol particle number concentrations and submicron particle mass loadings in many environments globally. Our recent investigations show that atmospheric NPF produces a significant amount of particles on days when no clear NPF event has been observed/identified. Furthermore, it has been observed in different environments all around the world that growth rates of nucleation mode particles vary little, usually much less than the measured concentrations of condensable vapors. It has also been observed that the local clustering, which in many cases acts as a starting point of regional new particle formation (NPF), can be described with the formation of intermediate ions at the smallest sizes. These observations, together with a recently developed ranking method, lead us to propose a paradigm shift in atmospheric NPF investigations. In this opinion paper, we will summarize the traditional approach of describing atmospheric NPF and describe an alternative method, covering both particle formation and initial growth. The opportunities and remaining challenges offered by the new approach are discussed.
- Article
(713 KB) - Full-text XML
- BibTeX
- EndNote
Atmospheric new particle formation (NPF) includes the formation of molecular clusters via different chemical pathways and the activation of some of these clusters for growth to larger sizes (Zhang et al., 2012; Kulmala et al., 2014; Lee et al., 2019; Kirkby et al., 2023). Depending on their subsequent fate in the atmosphere, essentially whether and how long they will survive from various sink processes (e.g., Kerminen et al., 2004; Pierce and Adams, 2007; Kulmala et al., 2017; Cai et al., 2022), these newly formed particles will contribute to the cloud condensation nuclei in a regional and global atmosphere (Spracklen et al., 2008; Wiedensohler et al., 2009; Kerminen et al., 2012; Gordon et al., 2017; Ren et al., 2021) and will act as seeds for haze particles during air pollution episodes in urban environments (e.g., Guo et al., 2014; Kulmala et al., 2022a).
In order to understand how atmospheric NPF influences climate and air quality and how these influences have changed over time or will change in the future as a result of anthropogenic and natural emission changes, we need to have detailed knowledge about the following issues in different atmospheric environments: (1) what the general characteristics of atmospheric NPF, including its frequency and intensity, are; (2) by which chemical mechanisms and constituents molecular clusters form and grow to larger sizes; and (3) how effectively newly formed particles reach sizes relevant to climate or air quality. In this opinion paper, we will focus on the first issue, acknowledging that the synergic effects of all of them need to be considered in order to get a full understanding of atmospheric NPF. We concentrate solely on field observations, as the power of laboratory experiments and model simulations is the strongest when investigating issues (2) and (3) mentioned above.
Traditionally, the general characteristics of atmospheric NPF have been investigated by concentrating on so-called NPF events, during which the formation of new particles has been so intense that a new mode of particles has been clearly observed. These events take place either locally close to emission sources of precursor compounds for this phenomenon or regionally over distances up to 1000 km or more (Kerminen et al., 2018, and references therein; Chu et al., 2019). With this approach, the particle concentrations resulting from atmospheric NPF can only be quantified for these clear NPF events, leaving little room for potentially low-intensity NPF in a regional atmosphere and providing practically no tools to handle local NPF. Another problem with the traditional approach is that the subsequent growth of newly formed particles to sizes relevant to climate or air quality can only be estimated for a small subset of cases, essentially those when both particle formation and growth take place relatively homogeneously in the regional atmosphere. In this opinion paper, we will propose an alternative approach to investigating atmospheric NPF, covering both particle formation and initial growth on all days with suitable aerosol data. We will discuss the opportunities that the new approach will offer for future investigations, as well as the remaining challenges, noting its complementary role when compared with traditional NPF event analysis and large-scale atmospheric model simulations.
In this section, we shortly discuss the approach traditionally used to investigate atmospheric NPF, including the history leading to this approach and its weaknesses. Based on our very recent work and findings, we then propose an alternative approach to tackle the problem, which may lead to a paradigm shift in investigating the general characteristics of atmospheric new particle formation using field observations. The main features of both approaches are summarized in Figs. 1 and 2 and are discussed in more detail in the following sections.
2.1 Traditional approach and associated shortcomings
Before continuous field observations, our understanding of atmospheric NPF relied entirely on theories and laboratory experiments. The first steps of NPF were described using classical nucleation theories which predict a very high dependence of the particle formation rate, or the nucleation rate, on the concentrations of vapors participating in NPF (e.g., Doyle, 1961; Jaecker-Voirol and Mirabel, 1989; Kulmala et al., 1991; Vehkamäki et al., 2002; Gaman et al., 2005). For many years, in laboratory experiments that have been conducted, the binary water–sulfuric acid nucleation has been assumed to be the only atmospherically relevant NPF pathway, and early experiments on this system supported the high sensitivity of the nucleation rate to the gas-phase sulfuric acid concentration (e.g., Wyslouzil et al., 1991; Viisanen et al., 1997). As a consequence, atmospheric NPF was essentially thought to be an on–off phenomenon that occurred sporadically under specific atmospheric conditions, essentially at high sulfuric acid concentrations.
The first field measurements of atmospheric NPF were made for specific types of plumes, including power plant plumes, in which NPF did not reach a regional extent (see Kerminen et al., 2018, and references therein). Such measurements were campaign-based and thus lack a statistical view on how frequent and intense NPF was or whether the newly formed particles were able to grow into sizes relevant to climate or air quality, essentially particles larger than about 50–100 nm in diameter. Later field observations, based either on campaign-wise or more continuous measurements at fixed locations, made it possible to identify and characterize regional NPF (Mäkelä et al., 1997; Kulmala et al., 2004; Kerminen et al., 2018). While such observations have dramatically enhanced our understanding of atmospheric NPF, they suffered from instrumental limitations and the non-homogenous nature of air masses that reach the measurement sites. As a result, it became common practice to characterize regional NPF by first estimating the NPF event frequency at the measurement site (i.e., fraction of days showing clear NPF) using some NPF event classification criteria (e.g., Dal Maso et al., 2005; Kulmala et al., 2012; Dada et al., 2018) and then determining particle formation and growth rates for a relatively small subset of days (Fig. 1), essentially those being strong and homogenous enough to permit determination of these quantities (e.g., Nieminen et al., 2018; Chu et al., 2019; Kanawade et al., 2022).
The traditional approach to investigating and characterizing atmospheric NPF based on event classification has obvious drawbacks. First, there are days when NPF is either relatively weak or affected by air mass non-homogeneities or changing weather conditions. Such days are often classified as undefined days (e.g., Dal Maso et al., 2005; Kulmala et al., 2012), sometimes further divided into a small number of sub-categories (e.g., Buenrostro Mazon et al., 2009; Dada et al., 2018) or incorrectly classified as non-event days (Kulmala et al., 2022b). Long-term measurements indicate that these mislabeled days tend to constitute a large or even a dominant fraction of all the days (Asmi et al., 2011; Kyrö et al., 2014; Dada et al., 2017; Wang et al., 2017; Kalivitis et al., 2019; Salma and Nemeth, 2019). Further analyses are thus difficult for such days. Second, the traditional approach is limited in providing information about the spatial and temporal variability of regional NPF, especially when it comes to regional intensity. Third, this approach is practically unable to capture sub-regional-scale NPF.
Over the years, the expected on–off behavior of atmospheric NPF has not been borne out by observations. Since the first simultaneous measurements of NPF and gas-phase sulfuric acid concentration (Weber et al., 1995, 1996), it has become clear that the observed formation rate of new particles in the atmosphere often scales between the first and second power of the sulfuric acid concentration (Sihto et al., 2006; Kuang et al., 2008), a much weaker dependency than predicted by classical nucleation theories discussed above. Such relatively weak dependence of the particle formation rate on gas-phase concentrations of compounds participating in NPF has been observed for practically all the NPF pathways identified as relevant for the atmosphere (Paasonen et al., 2010; Lehtipalo et al., 2018; Yao et al., 2018; Brean et al., 2020; He et al., 2021; Yan et al., 2021; Kirkby et al., 2023). Combining these field and laboratory observations strongly indicates that atmospheric NPF requires additional vapors beyond sulfuric acid and occurs over much larger concentration ranges of its precursor compounds than what has been thought before. Kulmala et al. (2022b) developed a method by which one could detect and even quantify the intensity of NPF on days traditionally classified as non-event days. They showed that NPF indeed occurs on such days and termed it “quiet NPF” because this phenomenon does not produce a visible NPF event in a surface plot illustrating the time evolution of a particle number distribution over a single day. The overall contribution of quiet NPF to the total production of new atmospheric aerosol particles appears to be non-negligible (Kulmala et al., 2022b; Tammet et al., 2014).
Investigations on the growth of newly formed particles to larger sizes are usually based on a relatively small subset of days on which NPF clearly occurs at a measurement site (Fig. 1). The main reason for this is that the most commonly used methods for determining particle growth rates (GRs) are only applicable for days during which there is a clear new mode of particles present in the particle size distribution that can be followed for several hours and that the growth is minimally affected by changes in measured air masses (e.g., Dal Maso et al., 2005; Kulmala et al., 2012). There is a danger that this approach gives a biased view on GR associated with atmospheric NPF, including the average level, variability, and particle size dependency of GR. Another, very common assumption is that the GR is determined almost solely by condensation of low-volatile vapors onto newly formed particles. This view is challenged by two findings. First, the average values of GR have been observed to vary little, often less than a factor of 2–3, between different environments and even less between different sites within a certain type of an environment (e.g., Kerminen et al., 2018; Nieminen et al., 2018). Second, and perhaps more importantly, GR was found to depend weakly on concentrations of “known” low-volatility vapors at two entirely different sites: urban Beijing, China (Kulmala et al., 2022a), and SMEAR II station in boreal forest, Finland (Kulmala et al., 2023). Acknowledging that aerosol physical processes (coagulation and cluster collisions with growing particles) usually have minor influences on GR (Stolzenburg et al., 2023), this “growth mystery” has two apparent explanations: either (1) our understanding of the mixture of vapors effectively condensing onto small particles, or the associated thermodynamics, is fundamentally incomplete, or (2) more species of volatile atmospheric vapors contribute to GR, e.g., via chemical reactions on particle surfaces (see detailed discussion in Kulmala et al., 2022a).
2.2 New, alternative approach and new opportunities
Instead of separately estimating the frequency of NPF and its intensity for a subset of days, often comprising only a small fraction of all days at any individual site, we propose that these two quantities will be combined into a probability distribution of the intensity of NPF that in practice covers all the days (Fig. 2). The intensity of NPF would in this case mean the formation rates of new particles, JC, at some fixed particle diameter, dC. Provided that the particle growth rate at sizes close to or slightly above dC is known, or can be estimated, the values of JC can be determined in the same way as in the traditional NPF event analysis (e.g., Kulmala et al., 2012) and then integrated over a desired period of time (daily, sub-daily, or instantaneous values). To get the best benefit of the derived distribution of JC for subsequent application purposes, the chosen value of dC should be large enough so that the complicated and poorly understood processes that determine survival probabilities of growing clusters and particles would mainly be restricted to sizes below dC (see, e.g., Kulmala et al., 2017). According to our current understanding in this respect (Cai et al., 2022; Tuovinen et al., 2022), dC should be 3 nm minimum and preferably somewhat larger under heavily polluted conditions. The upper limit of dC should be selected so that the calculated values of JC would be minimally affected by primary particle sources. While this is of little concern in remote or most rural areas, fresh primary particles, e.g., from traffic emission, are known to extend in size well below 10 nm (e.g., Rönkkö et al., 2017).
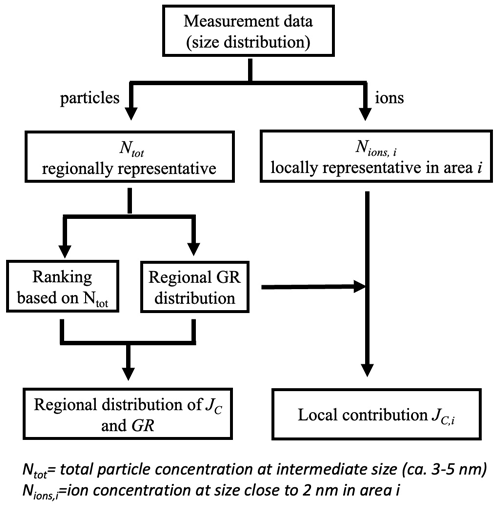
Figure 2Schematics of the new method proposed in this paper to characterize both regional and local NPF.
An immediate question is how to determine the probability distribution of the particle formation rate, especially at the lower end of this distribution that represents weak to moderate NPF. Kulmala et al. (2022b) demonstrated that by averaging and suitably scaling over a large number of measurement days, it is possible to estimate particle formation rates on days previously classified as non-event days using the traditional NPF event classification methods. However, this is not the only available option. Previous analyses have shown that atmospheric NPF is strongly associated with concentrations of intermediate ions, i.e., ions in the size range from 2 nm to a few nanometers (e.g., Tammet et al., 2014; Leino et al., 2016). Motivated by this finding, we recently investigated the sensitivity of the total particle number concentration in that size range to NPF using long-term measurement data from the SMEAR II station in Finland (Aliaga et al., 2023). We found that the days with higher 2.5–5 nm particle concentrations showed, on average, both higher particle formation rates and, in terms of traditional NPF event classification, higher probability of a NPF event to occur (Aliaga et al., 2023). Such a ranking method appears to be a promising candidate for creating a probability distribution of particle formation rates; however, its performance in different environments needs to be carefully tested. So far, besides the SMEAR II station, the preliminary results from a mountain site (Chacaltaya in Bolivia) and polluted sites (Beijing in China, Po Valley in Italy, El Alto in Bolivia) are promising.
Concerning particle growth, we propose that rather than only determining GR for a small subset of days, as usually done when analyzing field measurements, one should aim to find a relation between GR and the prevailing chemical environment. By a chemical environment we mean the presence (concentrations) of vapors that potentially contribute to GR and the activity of processes (condensation, heterogeneous reactions) that link these vapors to GR. We note that in any physical environment, its chemical environment determining GR may vary with time due to the variability in ambient temperature, solar radiation, and relatively humidity or due to changes in anthropogenic or biogenic emissions affecting this environment. Important to keep in mind when doing all this is that not only the least volatile vapors but also more volatile vapors capable of producing non-volatile vapors via heterogeneous reactions in and/or on particles may have a significant contribution to GR (e.g., Stolzenburg et al., 2023). To a first approximation, regionally representative values of GR and its variability could be derived using the largest subset of high rankings that display particle growth, even without detailed information needed to tie GR to the chemical environment. This approach can be justified by the fact that GR is determined by the prevailing chemical environment rather than the intensity of NPF; therefore, losing information from days with low-intensity NPF does not cause a serious bias in GR estimates (Kulmala et al., 2022b). It is also possible to improve the representativity of the GR for individual days, depending on the length of the observation data set, by investigating the typical GR in different seasons under different meteorological conditions or under otherwise varying situations in the chemical environment.
Analysis of both ions and particles in the 2–5 nm size range might provide a tool to combine regional and sub-regional NPF into the same framework. Using measurement data from the SMEAR II station and performing a theoretical analysis, it was demonstrated that the concentration of negative ions in a narrow size range of 2.0–2.3 nm could be related to the intensity of NPF averaged over a spatial scale of the order 1 km surrounding the measurement site (Tuovinen et al., 2024; Kulmala et al., 2024). If this is more generally applicable, including other sites with differing molecular particle forming mechanisms, targeted measurements of 2.0–2.3 nm ions could thereby be applied for identifying, and possibly quantifying, how effectively a specific (local) environment will produce new particles in the atmosphere (Fig. 2). Although such measurements say nothing about the subsequent fate of these particles, to a first approximation we may assume that they will grow essentially in the same manner as any newly formed in the same regional atmosphere or, more specifically, in the same prevailing chemical environment mentioned above. We cannot go to sizes smaller than 2 nm, since concentrations and dynamics of smaller ions are determined by processes which have very little to do with NPF (e.g., Tammet et al., 2014).
Based on these recent results and the new reasoning presented above, we suggest the following paradigm shift when investigating the general characteristics of atmospheric NPF using field measurements (see also Fig. 2).
-
Instead of doing binary (event, non-event) classification of NPF, we will utilize all days in the analysis and use a more continuous approach, such as the ranking method, for statistical information on the intensity of NPF.
-
We use particle and ion number concentrations in the smallest possible size regimes:
- a.
total particles (2.5–5 nm) or intermediate ions (2–7 nm) to study regional NPF
- b.
ions at diameters as close to 2 nm as possible to study local NPF.
- a.
-
We use the regionally representative particle growth rates, derived from the largest possibly subset of data, to calculate the following:
- a.
regional values of particle formation rates at selected sizes (3–5 nm) and integrated over desired time periods (instantaneous to daily)
- b.
local particle formation rates over selected areas and their relative contributions to regional NPF.
- a.
-
The particle formation rates can be determined for all days, and their distribution can be given as a continuous function of different parameters.
The main advance over the traditional method is that the new paradigm provides estimates of particle formation rates for all measurement days and in principle even continuous values as a function of time. But it remains to be investigated what the best time resolution is for doing this analysis in practice. This, together with regionally representative particle growth rates, provides us with a tool to quantify the contributions of both local and regional NPF to total particle number concentrations in a regional atmosphere.
Despite its highly promising potential to investigate atmospheric NPF, the new paradigm faces apparent challenges as well. For example, while continuous aerosol size distribution measurements are being conducted in several locations worldwide (e.g., Rose et al., 2021), a dominant fraction of these sites do not currently have proper instrumentation, such as NAIS (neutral cluster and air ion spectrometer), PSM (particle size magnifier) and nano-DMPS (nano-differential mobility particle size spectrometer) devices, for measuring the sub-5 nm size range needed for applying the alternative approach introduced here. The lack of available measurement is even more severe for ion measurements necessary for determining local or sub-regional NPF.
Dealing with primary emissions has been found to be difficult when investigating regional NPF in polluted environments (e.g., Woo et al., 2001; Ahlm et al., 2012; Nemeth et al., 2018; Pushpawela et al., 2018; Zhou et al., 2020; Kanawade et al., 2022), and the new, alternative approach introduced here is not expected to be free from the influences of primary emissions. Recently, methods have been developed to estimate, and potentially exclude, primary emissions originating from emissions, e.g., traffic (Okuljar et al., 2021; Chen et al., 2023), but the suitability of these methods for this proposed new approach remains to be investigated.
Particle survival probabilities are sensitive to the combined effect of the degree of pollution and particle growth rate, and there are large uncertainties in predicting this quantity in the sub-3 to 5 nm size range, especially in polluted environments (e.g., Kulmala et al., 2017; Cai et al., 2022; Tuovinen et al., 2022). This feature does not cause a major problem for investigating regional NPF, as long as the probability distribution of the particle formation rate is derived at large enough sizes, preferably at 5 nm and at 3 nm minimum. Concerning sub-regional NPF, we need to investigate whether and how particle survival probabilities influence the connection between ion concentrations close to 2 nm and particle formation rates at larger sizes, more specifically how the survival of sub-5 nm particles depends on their growth rate and background particle loading (condensation sink) in the environment considered (e.g., Tuovinen et al., 2022).
It is well known that both the occurrence and intensity of NPF vary seasonally at most of the sites (Dall'Osto et al., 2018; Kerminen et al., 2018; Nieminen et al., 2018; Chu et al., 2019, Brean et al., 2023). The potentially large seasonal variability of the mixture and concentrations of vapors contributing to particle formation and growth needs to be kept in mind when calculating particle formation and growth rates and when determining representative distributions for these quantities.
Finally, although the tools introduced here provide a first-order estimate of particle growth rates in different environments, we are far from a full understanding of which vapors and processes determine GR in different environmental conditions. As a result, much future work is needed to define, characterize, and quantify the chemical regimes and processes that eventually determine GR and its variability and how this variability feeds back into estimating particle formation rates during low-intensity NPF.
In this opinion, we have proposed a new method/approach and elucidated a paradigm shift in investigating atmospheric NPF using field observations. Contrary to the traditional event-based classification of individual days, the new approach looks at atmospheric NPF in a more statistical sense, aiming to create a probability distribution of particle formation and growth rates for all the days from continuous measurements at individual sites. While generally applicable to regional NPF, we also present ideas on how this same framework could be extended to sub-regional, or local, NPF.
The new approach provides a method to quantitatively estimate the contribution of atmospheric NPF to particle number concentration budgets in a regional atmosphere. If supported by additional measurements in areas with distinct sources for NPF precursors, the relative contributions of such source areas to the regional NPF can, in principle, be estimated. The results from the new approach can be extended to continental scales, provided that continuous measurement data from different representative regions are available.
The approach proposed here should be thought as complementary to the traditional NPF event analysis and large-scale model simulations. The traditional NPF event analysis has been widely used in the past, so its application to an entirely new data set offers a simple way to form ideas on how important NPF is in that particular environment and how it compares to other environments investigated earlier. Traditional NPF event analysis remains a powerful tool to select cases (days) for some special investigation purposes, such as investigating atmospheric NPF pathways and associated precursor chemistry associated with atmospheric NPF. The large-scale view on atmospheric NPF, including its climatic and health effects, as well as the associated feedback processes, has relied almost entirely on model simulations in the past. The proposed approach brings atmospheric measurements of NPF closer to results from large-scale model simulations, and, at the very least, the new paradigm offers an improved way to utilize measurement data to constrain and evaluate models simulating atmospheric NPF.
No data sets were used in this article.
MK and VMK had the original idea for the opinion paper. YC, AD, and DRW contributed to developing the idea further. VMK, MK, KL, and PP wrote the first version of the paper. DA, ST, RC, CY, FB, TP, HJ, VMK, and MK developed the material and results behind the opinion paper. All coauthors contributed to the final version of the paper.
At least one of the (co-)authors is a member of the editorial board of Aerosol Research. The peer-review process was guided by an independent editor, and the authors also have no other competing interests to declare.
Publisher’s note: Copernicus Publications remains neutral with regard to jurisdictional claims made in the text, published maps, institutional affiliations, or any other geographical representation in this paper. While Copernicus Publications makes every effort to include appropriate place names, the final responsibility lies with the authors.
Support from the technical and scientific staff in Hyytiälä and BUCT/AHL is acknowledged.
This research has been supported by the Research Council of Finland (grant nos. 337549, 302958, 325656, 311932, 334792, 316114, 325647, 325681, 333397, 328616, 357902, 345510, and 347782); the European Research Council, H2020 program (grant no. 742206); the Jane and Aatos Erkko Foundation (project: Quantifying carbon sink, CarbonSink+ and their interaction with air quality); the Jenny and Antti Wihuri Foundation (project: Gigacity); the European Union (projects: Non-CO2 Forcers and their Climate, Weather, Air Quality and Health Impacts (FOCI), CRiceS (grant no. 101003826), RI-URBANS (grant no. 101036245), EMMECARE (grant no. 856612), and FORCeS (grant no. 821205)); the Technology Industries of Finland Centennial Foundation (project: urbaani ilmanlaatu 2.0), University of Helsinki (ACTRIS-HY); the Estonian Research Council (project: PRG714); and the Estonian Environmental Observatory (KKOBS; project 2014-2020.4.01.20-0281).
This paper was edited by Daniele Contini and reviewed by two anonymous referees.
Ahlm, L., Liu, S., Day, D. A., Russell, L. M., Weber, R., Gentner, D. R., Goldstedin, A. H., Di Gangi, J. P., Henry, S. B., Keutsch, F. N., Van den Boer, T. C., Markovic, M. Z., Murphy, J. G., Ren, X., and Scheller, S.: Formation and growth of ultrafine particles from secondary sources in Bakersfield, California, J. Geophys. Res., 117, D00V08, https://doi.org/10.1029/2011JD017144, 2012.
Aliaga, D., Tuovinen, S., Zhang, T., Lampilahti, J., Li, X., Ahonen, L., Kokkonen, T., Nieminen, T., Hakala, S., Paasonen, P., Bianchi, F., Worsnop, D., Kerminen, V.-M., and Kulmala, M.: Nanoparticle ranking analysis: determining new particle formation (NPF) event occurrence and intensity based on the concentration spectrum of formed (sub-5 nm) particles, Aerosol Research, 1, 81–92, https://doi.org/10.5194/ar-1-81-2023, 2023.
Asmi, E., Kivekäs, N., Kerminen, V.-M., Komppula, M., Hyvärinen, A.-P., Hatakka, J., Viisanen, Y., and Lihavainen, H.: Secondary new particle formation in Northern Finland Pallas site between the years 2000 and 2010, Atmos. Chem. Phys., 11, 12959–12972, https://doi.org/10.5194/acp-11-12959-2011, 2011.
Brean, J., Beddows, D. C. S., Shi, Z., Temime-Roussel, B., Marchand, N., Querol, X., Alastuey, A., Minguillón, M. C., and Harrison, R. M.: Molecular insights into new particle formation in Barcelona, Spain, Atmos. Chem. Phys., 20, 10029–10045, https://doi.org/10.5194/acp-20-10029-2020, 2020.
Brean, J., Beddows, D. C. S., Harrison, R. M., Song, C., Tunved, P., Ström, J., Krejci, R., Freud, E., Massling, A., Skov, H., Asmi, E., Lupi, A., and Dall'Osto, M.: Collective geographical ecoregions and precursor sources driving Arctic new particle formation, Atmos. Chem. Phys., 23, 2183–2198, https://doi.org/10.5194/acp-23-2183-2023, 2023.
Buenrostro Mazon, S., Riipinen, I., Schultz, D. M., Valtanen, M., Dal Maso, M., Sogacheva, L., Junninen, H., Nieminen, T., Kerminen, V.-M., and Kulmala, M.: Classifying previously undefined days from eleven years of aerosol-particle-size distribution data from the SMEAR II station, Hyytiälä, Finland, Atmos. Chem. Phys., 9, 667–676, https://doi.org/10.5194/acp-9-667-2009, 2009.
Cai, R., Deng, C., Stolzenburg, D., Li, C., Guo, J., Kerminen, V.-M., Jiang, J., Kulmala, M., and Kangasluoma, J.: Survival probability of new atmospheric particles: closure between theory and measurements from 1.4 to 100 nm, Atmos. Chem. Phys., 22, 14571–14587, https://doi.org/10.5194/acp-22-14571-2022, 2022.
Chen, L., Qi, X., Niu, G., Li, Y., Liu, C., Lai, S., Liu, Y., Nie, W., Yan, C., Wang, J., Chi, X., Paasonen, P., Hussein, T., Lehtipalo, K., Kerminen, V.-M., Petäjä, T., Kulmala, M., and Ding, A.: High concentration of atmospheric sub-3 nm particles in polluted environment of eastern China: New particle formation and traffic emission. J. Geophys. Res.-Atmos., 128, e2023JD039669, https://doi.org/10.1029/2023JD039669, 2023.
Chu, B., Kerminen, V.-M., Bianchi, F., Yan, C., Petäjä, T., and Kulmala, M.: Atmospheric new particle formation in China, Atmos. Chem. Phys., 19, 115–138, https://doi.org/10.5194/acp-19-115-2019, 2019.
Dada, L., Paasonen, P., Nieminen, T., Buenrostro Mazon, S., Kontkanen, J., Peräkylä, O., Lehtipalo, K., Hussein, T., Petäjä, T., Kerminen, V.-M., Bäck, J., and Kulmala, M.: Long-term analysis of clear-sky new particle formation events and nonevents in Hyytiälä, Atmos. Chem. Phys., 17, 6227–6241, https://doi.org/10.5194/acp-17-6227-2017, 2017.
Dada, L., Chellapermal, R., Buenrostro Mazon, S., Paasonen, P., Lampilahti, J., Manninen, H. E., Junninen, H., Petäjä, T., Kerminen, V.-M., and Kulmala, M.: Refined classification and characterization of atmospheric new-particle formation events using air ions, Atmos. Chem. Phys., 18, 17883–17893, https://doi.org/10.5194/acp-18-17883-2018, 2018.
Dall'Osto, M., Beddows, D. C. S., Asmi, A., Poulain, L., Hao, L, Freney, E., Allan, J. D., Canagaratna, M, Crippa, M., Bianchi, F., de Leeuw, G., Eriksson, A., Swietlicki, E., Hansson, H. C., Heinzing, J. S., Cranier, C., Zemankova, K., Laj, P., Onasch, T., Prevot, A., Putaud, J. P., Sellegri, K., Vidal, M., Virtanen, A., Simo, R., Worsnop, D., O'Dowd, C., Kulmala, M., and Harrison, R. M.: Novel insights on new particle formation from a pan-european observing system, Sci. Rep., 8, 1482, https://doi.org/10.1038/s41598-017-17343-9, 2018.
Dal Maso, M., Kulmala, M., Riipinen, I., Wagner, R., Hussein, T., Aalto, P. P., and Lehtinen, K. E. J.: Formation and growth of fresh atmospheric aerosols: eight years of aerosol size distribution data from SMEAR II, Hyytiälä, Finland, Boreal Environ. Res., 10, 323–336, 2005.
Doyle, G. J.: Self-nucleation in the sulfuric acid-water system, J. Chem. Phys., 35, 795–799, https://doi.org/10.1063/1.1701218, 1961.
Gaman, A., Napari, I., Winkler, P. M., Vehkamäki, H., Wagner, P. E., Strey, R., Viisanen, Y., and Kulmala, M.: Homogeous nucleation of n-nonane and n-propano mixtures: A comparison of classical nucleation theory and experiments, J. Chem. Phys., 123, 244502, https://doi.org/10.1063/1.2138703, 2005.
Gordon, H., Kirkby, J., Baltensperger, U., Bianchi, F., Breitenlechner, M., Curtius, J., Dias, A., Dommen, J., Donahue, N. M., Dunne, E. M., Duplissy, J., Ehrhart, S., Flagan, R. C., Frege, C., Fuchs, C., Hansel, A., Hoyle, C. R., Kulmala, M., Kürten, A., Lehtipalo, K., Makhmutov, V., Molteni, U., Rissanen, M. P., Stozhov, Y., Tröstl, J., Tsakogeorgas, G., Wagner, R., Williamson, C., Wimmer, D., Winkler, P. M., Yan, C., and Carslaw, K. S.: Causes and importance of new particle formation in the present-day and preindustrial atmospheres. J. Geophys. Res.-Atmos., 122, 8739–8760, https://doi.org/10.1002/2017JD026844, 2017.
Guo, S., Hu, M., Zamora, M. L., Peng, J., Shang, D., Zheng, J., Du, Z., Wu, Z., Shao, M., Zeng, L., Molina, M. J., and Zhang, R.: Elucidating severe urban haze formation in China, P. Natl. Acad. Sci. USA, 111, 17373, https://doi.org/10.1073/pnas.1419604111, 2014.
He, X.-C., Tham, Y. J., Dada, L., Wang, M., Finkenzeller, H.,Stolzenburg, D., Iyer, S. Simon, M., Kürten, A., Shen, J., Rörup, B., Rissanen, M., Schobesberger, S., Baalbaki, Wang, D. S., Koenig, T. K., Jokinen, T., Sarnela, N. Beck, L., Almeida, J., Amanatidis, S., Amorim1, A., Ataei, F., Baccarini, A., Bertozzi, B., Bianchi, F., Brilke, S., Caudillo, L., Chen, D., Chiu, R., Chu, B., Dias, A., Ding, A., Dommen, J., Duplissy, J., El Haddad, I., Carracedo, L. G., Granzin, M., Hansell, A., Heinritzi, M.,Hofbauer, V., Junninen, H., Kangasluoma, J., Kemppainen, D., Kim, C., Kong, W., Krechmer, J. E., Kvashin, A., Laitinen, T., Lamkaddam, H., Lee, C. P., Lehtipalo, K., Leiminger, M., Li, Z., Makhmutov, V., Manninen, H. E., Marie, G., Marten, R., Mathot, S., Mauldin, R. L., Mentler, B. Möhler, O., Müller, T., Nie, W., Onnela, A., Petäjä, T., Pfeifer, J., Philippov, M., Ranjithkumar, A., Saiz-Lopez, A., Salma, I., Scholz, W., Schuchmann, S., Schulze, B., Steiner, G., Stozhkov, Y., Tauber, C., Tomé, A., Thakur, R. C., Väisänen, O., Vazquez-Pufleau, M., Wagner, A. C., Wang, Y., Weber, S. K., Winkler, P. M., Wu, Y., Xiao, M., Yan, C., Ye, Q., Ylisirniö, A., Zauner-Wieczorek, M., Zha, Q., Zhou, P., Flagan, R. C., Curtius, J., Baltensperger, U., Kulmala, M., Kerminen, V.-M., Kurtén, T., Donahue, N. M., Volkamer, R., Kirkby, J., Worsnop, D. R., and Sipilä, M.: Role of iodine oxoacids in atmospheric aerosol nucleation, Science, 371, 589–595, https://doi.org/10.1126/science.abe0298, 2021.
Jaecker-Voirol, A. and Mirabel, P.: Heteromolecular nucleation in the sulfuric acid-water system, Atmos. Environ., 23, 2053–2057, https://doi.org/10.1016/0004-6981(89)90530-1, 1989.
Kalivitis, N., Kerminen, V.-M., Kouvarakis, G., Stavroulas, I., Tzitzikalaki, E., Kalkavouras, P., Daskalakis, N., Myriokefalitakis, S., Bougiatioti, A., Manninen, H. E., Roldin, P., Petäjä, T., Boy, M., Kulmala, M., Kanakidou, M., and Mihalopoulos, N.: Formation and growth of atmospheric nanoparticles in the eastern Mediterranean: results from long-term measurements and process simulations, Atmos. Chem. Phys., 19, 2671–2686, https://doi.org/10.5194/acp-19-2671-2019, 2019.
Kanawade, V. J., Sebastian, M., Hooda, R. K., and Hyvärinen, A.-P.: Atmospheric new particle formation in India: current understanding and knowledge gaps, Atmos. Environ., 270, 118894, https://doi.org/10.1016/j.atmosenv.2021.118894, 2022.
Kerminen, V.-M., Lehtinen, K. E. J., Anttila, T., and Kulmala, M.: Dynamics of atmospheric nucleation mode particles: a time scale analysis, Tellus, 56B, 135–146, https://doi.org/10.3402/tellusb.v56i2.16411, 2004.
Kerminen, V.-M., Paramonov, M., Anttila, T., Riipinen, I., Fountoukis, C., Korhonen, H., Asmi, E., Laakso, L., Lihavainen, H., Swietlicki, E., Svenningsson, B., Asmi, A., Pandis, S. N., Kulmala, M., and Petäjä, T.: Cloud condensation nuclei production associated with atmospheric nucleation: a synthesis based on existing literature and new results, Atmos. Chem. Phys., 12, 12037–12059, https://doi.org/10.5194/acp-12-12037-2012, 2012.
Kerminen, V.-M., Chen, X., Vakkari, V., Petäjä, T., Kulmala, M., and Bianchi, F.: Atmospheric new particle formation and growth: review of field observations, Environ. Res. Lett., 13, 103003, https://doi.org/10.1088/1748-9326/aadf3c, 2018.
Kirkby, J., Amorim, A., Baltensperger, U., Carslaw, K. S., Christoudias, T., Curtius, J., Donahue, N. M., El Haddad, I., Flagan, R. C., Gordon, H., Hansel, A., Harder, H., Junninen, H., Kulmala, M., Kurten, A., Laaksonen, A., Lehtipalo, K., Lelieveld, J., Mohler, O., Riipinen, I., Stratmann, F., Tome, A., Virtanen, A., Volkamer, R., Winkler, P. M., and Worsnop, D. R.: Atmospheric new particle formation from the CERN CLOUD experiment, Nat. Geosci., 16, 948–957, https://doi.org/10.1038/s41561-023-01305-0, 2023.
Kuang, C., McMurry, P. H., McCormick, A. V., and Eisele, F., L.: Dependence of nucleation rates on sulfuric acid vapor concentration in diverse atmospheric locations, J. Geophys. Res., 113, D10209, https://doi.org/10.1029/2007JD009253, 2008.
Kulmala, M., Laaksonen, A., and Jokiniemi, J.: Numerical simulations of binary nucleation of hydrogen iodide and water vapors, J. Aerosol Sci., 22, 149–157, https://doi.org/10.1016/0021-8502(91)90024-C, 1991.
Kulmala, M., Vehkamäki, H., Petäjä, T., Dal Maso, M., Lauri, A., Kerminen, V.-M., Birmili, W., and McMurry, P. H.: Formation and growth rates of ultrafine atmospheric particles: A review of observations, J. Aerosol Sci., 35, 143–176, https://doi.org/10.1016/j.jaerosci.2003.10.003, 2004.
Kulmala, M., Petäjä, T., Nieminen, T., Sipilä, M., Manninen, H. E., Lehtipalo, K., Dal Maso, M., Aalto, P. P., Junninen, H., Paasonen, P., Riipinen, I., Lehtinen, K. E. J., Laaksonen, A., and Kerminen, V.-M.: Measurement of the nucleation of atmospheric aerosol particles, Nat. Protoc., 7, 1651–1667, https://doi.org/10.1038/nprot.2012.091, 2012.
Kulmala, M., Petäjä, T., Ehn, M., Thornton, J., Sipilä, M., Worsnop, D. R., and Kerminen, V.-M.: Chemistry of atmospheric nucleation: On the recent advances on precursor characterization and atmospheric cluster composition in connection with atmospheric new particle formation, Annu. Rev. Phys. Chem., 65, 21–37, https://doi.org/10.1146/annurev-physchem-040412-110014, 2014.
Kulmala, M., Kerminen, V.-M., Petäjä, T., Ding, A. J., and Wang, L.: Atmospheric gas-to-particle conversion: why NPF events are observed in megacities?, Faraday Discuss., 200, 271–288, https://doi.org/10.1039/C6FD00257A, 2017.
Kulmala, M., Cai, R., Stolzenburg, D., Zhou, Y., Dada, L., Guo, Y., Yan, C., Petäjä, T., Jiang, J., and Kerminen, V.-M.: The contribution of new particle formation and subsequent growth to haze formation, Environ. Sci. Atmos., 2, 352–361, https://doi.org/10.1039/D1EA00096A, 2022a.
Kulmala, M., Junninen, H., Dada, L., Salma, I., Weidinger, T., Thén, W., Vörösmarty, M., Komsaare, K., Stolzenburg, D., Cai, R., Yan, C., Li, X., Deng, C., Jiang, J., Petäjä, T., Nieminen, T., and Kerminen, V.-M.: Quiet new particle formation in the atmosphere. Front. Environ. Sci., 10, 912385, https://doi.org/10.3389/fenvs.2022.912385, 2022b.
Kulmala, M., Cai, R., Ezhova, E., Deng, C., Stolzenburg, D., Dada, L., Guo, Y., Yan, C., Peräkylä, O., Lintunen, A., Nieminen, T., Kokkonen, T. V., Sarnela, N., Petäjä, T., and Kerminnen, V.-M.: Direct link between the characteristics of atmospheric new particle formation and Continental Biosphere-Atmosphere-Cloud-Climate (COBACC) feedback loop, Boreal. Environ. Res., 28, 1–13, 2023.
Kulmala, M., Ke, P., Lintunen, A., Peräkylä, O., Lohtander, A., Tuovinen, S., Lampilahti, J., Kolari, P., Schiestl-Aalto, P., Kokkonen, T., Nieminen, T., Dada, L., Ylivinkka, I., Petäjä, T., Bäck, J., Lohila, A., Heimsch, L., Ezhova, E., and Kerminen, V.-M.: A novel concept for assessing the potential of different boreal ecosystems to mitigate climate change (CarbonSink+ Potential), Boreal Environ. Res., 29, 1–16, 2024.
Kyrö, E.-M., Väänänen, R., Kerminen, V.-M., Virkkula, A., Petäjä, T., Asmi, A., Dal Maso, M., Nieminen, T., Juhola, S., Shcherbinin, A., Riipinen, I., Lehtipalo, K., Keronen, P., Aalto, P. P., Hari, P., and Kulmala, M.: Trends in new particle formation in eastern Lapland, Finland: effect of decreasing sulfur emissions from Kola Peninsula, Atmos. Chem. Phys., 14, 4383–4396, https://doi.org/10.5194/acp-14-4383-2014, 2014.
Lee, S.-H., Gordon, H., Yu, H., Lehtipalo, K., Haley, R., Li, X., and Zhang, R.: New particle formation in the atmosphere: From molecular clusters to global climate, J. Geophys. Res.-Atmos., 124, 7098–7146, https://doi.org/10.1029/2018JD029356, 2019.
Lehtipalo, K., Yan, C., Dada, L., Bianchi, F., Xiao, M., Wagner, R., Stolzenburg, D., Ahonen, L. R., Amorim, A., Baccarini, A., Bauer, P. S., Baumgartner, B., Bergen, A., Bernhammer, A.-K., Breitenlechner, M., Brilke, S., Buckholz, A., Buenrostro Mazon, S., Chen, D., Chen, X., Dias, A., Dommen, J., Draper, D. C., Duplissy, J., Ehn, M., Finkenzeller, H., Fischer, L., Frege, C., Fuchs, C., Garmash, O., Gordon, H., Hakala, J., He, X., Heikkinen, L., Heinrizi, M., Helm, J. C., Hofbauer, V., Hoyle, C. R., Jokinen, T., Kangasluoma, J., Kerminen, V.-M., Kim, C., Kirkby, J., Kontkanen, J., Kürten, A., Lawler, M. J., Mai, H., Mathot, S., Mauldin, III R. L., Molteni, U., Nichman, L., Nie, W., Nieminen, T., Ojdanic, A., Onnela, A., Passananti, M., Petäjä, T., Piel, F., Pospisilova, V., Quéléver, L. L. J., Rissanen, M. P., Rose, C., Sarnela, N., Schallhart, S., Schuchmann, S., Sengupta, K., Simon, M., Sipilä, M., Tauber, K., Tomé, A., Tröstl, J., Väisänen, O., Vogel, A. L., Volkamer, A., Wagner, A. C., Wang, M., Weitz, L., Wimmer, D., Ye, P., Ylisirniö, A., Zha, Q., Carslaw, K. S., Curtius, J., Donahue, N. M., Flagan, R. C., Hansel, A., Riipinen, I., Virtanen, A., Winkler, P. M., Baltensperger, U., Kulmala, M., and Worsnop, D. R.: Multicomponenent new particle formation from sulfuric acid, ammonia, and biogenic vapors, Sci. Adv., 4, eaau5363, https://doi.org/10.1126/sciadv.aau5363, 2018.
Leino, K., Nieminen, T., Manninen, H. E., Petäjä, T., Kerminen, V.-M., and Kulmala, M.: Intermediate ions as a strong indicator of new particle formation bursts in boreal forest, Boreal Environ. Res., 21, 274–286, 2016.
Mäkelä, J. M., Aalto, P., Jokinen, V., Pohja, T., Nissinen, A., Palmroth, S., Markkanen, T., Seitsonen, K., Lihavainen, H., and Kulmala, M.: Observations of ultrafine aerosol particle formation and growth in boreal forest, Geophys. Res. Lett., 24, 1219–1222, https://doi.org/10.1029/97GL00920, 1997.
Nemeth, Z., Rosati, B., Zikova, N., Salma, I., Bozo, L., de Espana, C. D., Schwartz, J., Zdimal, V., and Wonaschutz, A.: Comparison of atmospheric new particle formation events in three Central European cities, Atmos. Environ., 178, 191–197, 2018.
Nieminen, T., Kerminen, V.-M., Petäjä, T., Aalto, P. P., Arshinov, M., Asmi, E., Baltensperger, U., Beddows, D. C. S., Beukes, J. P., Collins, D., Ding, A., Harrison, R. M., Henzing, B., Hooda, R., Hu, M., Hõrrak, U., Kivekäs, N., Komsaare, K., Krejci, R., Kristensson, A., Laakso, L., Laaksonen, A., Leaitch, W. R., Lihavainen, H., Mihalopoulos, N., Németh, Z., Nie, W., O'Dowd, C., Salma, I., Sellegri, K., Svenningsson, B., Swietlicki, E., Tunved, P., Ulevicius, V., Vakkari, V., Vana, M., Wiedensohler, A., Wu, Z., Virtanen, A., and Kulmala, M.: Global analysis of continental boundary layer new particle formation based on long-term measurements, Atmos. Chem. Phys., 18, 14737–14756, https://doi.org/10.5194/acp-18-14737-2018, 2018.
Okuljar, M., Kuuluvainen, H., Kontkanen, J., Garmash, O., Olin, M., Niemi, J. V., Timonen, H., Kangasluoma, J., Tham, Y. J., Baalbaki, R., Sipilä, M., Salo, L., Lintusaari, H., Portin, H., Teinilä, K., Aurela, M., Dal Maso, M., Rönkkö, T., Petäjä, T., and Paasonen, P.: Measurement report: The influence of traffic and new particle formation on the size distribution of 1–800 nm particles in Helsinki – a street canyon and an urban background station comparison, Atmos. Chem. Phys., 21, 9931–9953, https://doi.org/10.5194/acp-21-9931-2021, 2021.
Paasonen, P., Nieminen, T., Asmi, E., Manninen, H. E., Petäjä, T., Plass-Dülmer, C., Flentje, H., Birmili, W., Wiedensohler, A., Hõrrak, U., Metzger, A., Hamed, A., Laaksonen, A., Facchini, M. C., Kerminen, V.-M., and Kulmala, M.: On the roles of sulphuric acid and low-volatility organic vapours in the initial steps of atmospheric new particle formation, Atmos. Chem. Phys., 10, 11223–11242, https://doi.org/10.5194/acp-10-11223-2010, 2010.
Pierce, J. R. and Adams, P. J.: Efficiency of cloud condensation nuclei formation from ultrafine particles, Atmos. Chem. Phys., 7, 1367–1379, https://doi.org/10.5194/acp-7-1367-2007, 2007.
Pushpawela, B., Jayaratne, R., and Morawska, L.: Differentiating between particle formation and growth events in an urban environment, Atmos. Chem. Phys., 18, 11171–11183, https://doi.org/10.5194/acp-18-11171-2018, 2018.
Ren, J., Chen, L., Fan, T., Liu, J., Jiang, S., and Zhang, F.: The NPF effect on CCN number concentrations: a review and re-evaluation of observations from 35 sites worldwide, Geophys. Res. Lett., 48, e2021GL095190, https://doi.org/10.1029/2021GL095190, 2021.
Rose, C., Collaud Coen, M., Andrews, E., Lin, Y., Bossert, I., Lund Myhre, C., Tuch, T., Wiedensohler, A., Fiebig, M., Aalto, P., Alastuey, A., Alonso-Blanco, E., Andrade, M., Artíñano, B., Arsov, T., Baltensperger, U., Bastian, S., Bath, O., Beukes, J. P., Brem, B. T., Bukowiecki, N., Casquero-Vera, J. A., Conil, S., Eleftheriadis, K., Favez, O., Flentje, H., Gini, M. I., Gómez-Moreno, F. J., Gysel-Beer, M., Hallar, A. G., Kalapov, I., Kalivitis, N., Kasper-Giebl, A., Keywood, M., Kim, J. E., Kim, S.-W., Kristensson, A., Kulmala, M., Lihavainen, H., Lin, N.-H., Lyamani, H., Marinoni, A., Martins Dos Santos, S., Mayol-Bracero, O. L., Meinhardt, F., Merkel, M., Metzger, J.-M., Mihalopoulos, N., Ondracek, J., Pandolfi, M., Pérez, N., Petäjä, T., Petit, J.-E., Picard, D., Pichon, J.-M., Pont, V., Putaud, J.-P., Reisen, F., Sellegri, K., Sharma, S., Schauer, G., Sheridan, P., Sherman, J. P., Schwerin, A., Sohmer, R., Sorribas, M., Sun, J., Tulet, P., Vakkari, V., van Zyl, P. G., Velarde, F., Villani, P., Vratolis, S., Wagner, Z., Wang, S.-H., Weinhold, K., Weller, R., Yela, M., Zdimal, V., and Laj, P.: Seasonality of the particle number concentration and size distribution: a global analysis retrieved from the network of Global Atmosphere Watch (GAW) near-surface observatories, Atmos. Chem. Phys., 21, 17185–17223, https://doi.org/10.5194/acp-21-17185-2021, 2021.
Rönkkö, T., Kuuluvainen, H., Karjalainen, P., Keskinen, J., Hillamo, R., Niemi, J. V., Pirjola, L., Timonen, H. J., Saarikoski, S., Saukko, E., Järvinen, A., Silvennoinen, H., Rostedt, A., Olin, M., Yli-Ojanperä, J., Nousiainen, P., Kousa, A., and Dal Maso, M.: Traffic is a major source of atmospheric nanocluster aerosol, P. Natl. Acad. Sci. USA, 114, 7549–7554, https://doi.org/10.1073/pnas.1700830114, 2017.
Salma, I. and Németh, Z.: Dynamic and timing properties of new aerosol particle formation and consecutive growth events, Atmos. Chem. Phys., 19, 5835–5852, https://doi.org/10.5194/acp-19-5835-2019, 2019.
Sihto, S.-L., Kulmala, M., Kerminen, V.-M., Dal Maso, M., Petäjä, T., Riipinen, I., Korhonen, H., Arnold, F., Janson, R., Boy, M., Laaksonen, A., and Lehtinen, K. E. J.: Atmospheric sulphuric acid and aerosol formation: implications from atmospheric measurements for nucleation and early growth mechanisms, Atmos. Chem. Phys., 6, 4079–4091, https://doi.org/10.5194/acp-6-4079-2006, 2006.
Spracklen, D. V., Carslaw, K. S., Kulmala, M., Kerminen, V.-M., Sihto, S.-L., Riipinen, I., Merikanto, J., Mann, G. W., Chipperfield, M. P., Wiedensohler, A., Birmili, W., and Lihavainen, H.: Contribution of particle formation to global cloud condensation nuclei concentrations, Geophys. Res. Lett., 35, L06808, https://doi.org/10.1029/2007GL033038, 2008.
Stolzenburg, D., Cai, R., Blichner, S., Kontkanen, J., Zhou, P., Makkonen, R., Kerminen, V.-M., Kulmala, M., Riipinen, I., and Kangasluoma, J.: Atmospheric nanoparticle growth, Rev. Mod. Phys., 95, 045002, https://doi.org/10.1103/RevModPhys.95.045002, 2023.
Tammet, H, Komsaare, K., and Horrak, U.: Intermediate ions in the atmosphere, Atmos. Res., 135–136, 263–273, https://doi.org/10.1016/j.atmosres.2012.09.009, 2014.
Tuovinen, S., Cai, R., Kerminen, V.-M., Jiang, J., Yan, C., Kulmala, M., and Kontkanen, J.: Survival probabilities of atmospheric particles: comparison based on theory, cluster population simulations, and observations in Beijing, Atmos. Chem. Phys., 22, 15071–15091, https://doi.org/10.5194/acp-22-15071-2022, 2022.
Tuovinen, S., Lampilahti, J., Kerminen, V.-M., and Kulmala, M.: Intermediate ions as indicator for local new particle formation, Aerosol Research Discuss. [preprint], https://doi.org/10.5194/ar-2024-4, in review, 2024.
Vehkamäki, H., Kulmala, M., Napari, I., Lehtinen, K. E. J., Timmreck, C., Noppel, M., and Laaksonen, A.: An improved parameterization for sulfuric acid-water nucleation rates for tropospheric and stratospheric conditions. J. Geophys. Res., 107, 4622, https://doi.org/10.1029/2002JD002184, 2002.
Viisanen, Y., Kulmala, M., and Laaksonen, A.: Experiments on gas-liquid nucleation of sulfuric acid and water, J. Chem. Phys., 107, 920–926, https://doi.org/10.1063/1.474445, 1997.
Wang, Z., Birmili, W., Hamed, A., Wehner, B., Spindler, G., Pei, X., Qu, Z., Cheng, Y., Su, H., and Wiedensohler, A.: Contributions of volatile and nonvolatile compounds (at 300 °C) to condensational growth of atmospheric nanoparticles: An assessment based on 8.5 years of observations at the Central Europe background site Melpitz. J. Geophys. Res.-Atmos., 122, 485–497, https://doi.org/10.1002/2016JD025581, 2017.
Weber, R. J., McMurry, P. H., Eisele, F. L., and Tanner, D. J:, Measurement of expected nucleation precursor species and 3 to 500 nm particles at Mauna Loa Observatory, Hawaii, J. Atmos. Sci., 52, 2242–2257, https://doi.org/10.1175/1520-0469(1995)052<2242:MOENPS>2.0.CO;2, 1995.
Weber, R. J., Marti, J. J., McMurry, P. H., Eisele, F. L., Tanner, D. J., and Jefferson A.: Measured atmospheric new particle formation rates: Implications for nucleation mechanisms, Chem. Eng. Commun., 151, 53–64, https://doi.org/10.1080/00986449608936541, 1996.
Wiedensohler, A., Cheng, Y., Nowak, A., Wehner, B., Achtert, P. Berghof, M., Birmili, W., Wu, Z. J., Hu, M., Zhu, T., Takegawa, N., Kita, K., Kondo, J., Lou, S. R., Hofzumahaus, A., Holland, F., Wahner, A., Gunthe, S. S., Rose, D., Su, H., and Pöschl, U.: Rapid aerosol particle growth and increase of cloud condensation nucleus activity by secondary aerosol formation and condensation: a case study for regional air pollution in northeastern China, J. Geophys. Res.-Atmos., 114, D00G08, https://doi.org/10.1029/2008JD010884, 2009.
Woo, K. S., Chen, D. R., Pui, D. Y. H., and McMurry, P. H.: Measurement of Atlanta size distributions: observations of ultrafine particle formation events, Aerosol Sci. Tech., 34, 75–87, https://doi.org/10.1080/02786820120056, 2001.
Wyslouzil, B. E., Seinfeld, J. H., Flagan, R. C., and Okuyama, K.: Binary nucleation in acid-water and a comparison with methanesulfonic acid-water, J. Chem. Phys., 94, 6842–6850, https://doi.org/10.1063/1.460262, 1991.
Yan, C., Yin, R., Lu, Y., Dada, L., Yang, D., Fu, Y., Kontkanen, J., Deng, C., Garmash, O., Ruan, J., Baalbaki, R., Schervish, M., Cai, R., Bloss, M., Chan, T., Chen, T., Chen, Q., Chen, X., Chen, Y., Chu, B., Dällenbach, K., Foreback, B., He, X., Heikkinen, L., Jokinen, T., Junninen, H., Kangasluoma, J., Kokkonen, T., Kurppa, M., Lehtipalo, K., Li, H., Li, H., Li, X., Liu, Y. Ma, Q., Paasonen, P., Rantala, P., Pileci, R. E., Rusanen, A., Sarnela, N., Simonen, P., Wang, S., Wang, W., Wang, Y. Xue, M., Yang, G., Yao, L., Zhou, Y., Kujansuu, J., Petäjä, T., Nie, W., Ma, N., Ge, M., He, H., Donahue, N. M., Worsnop, D. R., Kerminen, V.-M., Wang, L., Liu, Y., Zheng, J., Kulmala, M., Jiang, J., and Bianchi, F.: The synergistic role of sulfuric acid, bases, and oxidized organics governing new-particle formation in Beijing, Geophys. Res. Lett., 48, e2020GL091944 , https://doi.org/10.1029/2020GL091944, 2021.
Yao, L., Garmash, O., Bianchi, F., Zhen, J., Yan, C., Kontkanen, J., Junninen, H., Buenrostro Mazon, S., Ehn, M., Paasonen, P., Sipilä, M., Wang, M., Wang, X., Xiao, S., Chen, H., Lu, Y., Zhang, B., Wang, D., Fu, Q., Geng, F., Li, L, Wang, H., Qiao, L., Yang, X, Chen, J., Kerminen, V.-M., Petäjä, T., Worsnop, D. R., Kulmala, M., and Wang, L.: Atmospheric new particle formation from sulfuric acid and amines in a Chinese megacity, Science, 361, 278–281, https://doi.org/10.1126/science.aao4839, 2018.
Zhang, R., Khalizov, A., Wang, L., Hu, M., and Xu, W.: Nucleation and growth in the atmosphere, Chem. Rev., 112, 1957–2011, https://doi.org/10.1021/cr2001756, 2012.
Zhou, Y., Dada, L., Liu, Y., Fu, Y., Kangasluoma, J., Chan, T., Yan, C., Chu, B., Daellenbach, K. R., Bianchi, F., Kokkonen, T. V., Liu, Y., Kujansuu, J., Kerminen, V.-M., Petäjä, T., Wang, L., Jiang, J., and Kulmala, M.: Variation of size-segregated particle number concentrations in wintertime Beijing, Atmos. Chem. Phys., 20, 1201–1216, https://doi.org/10.5194/acp-20-1201-2020, 2020.